How To Find Energy Levels Of An Element
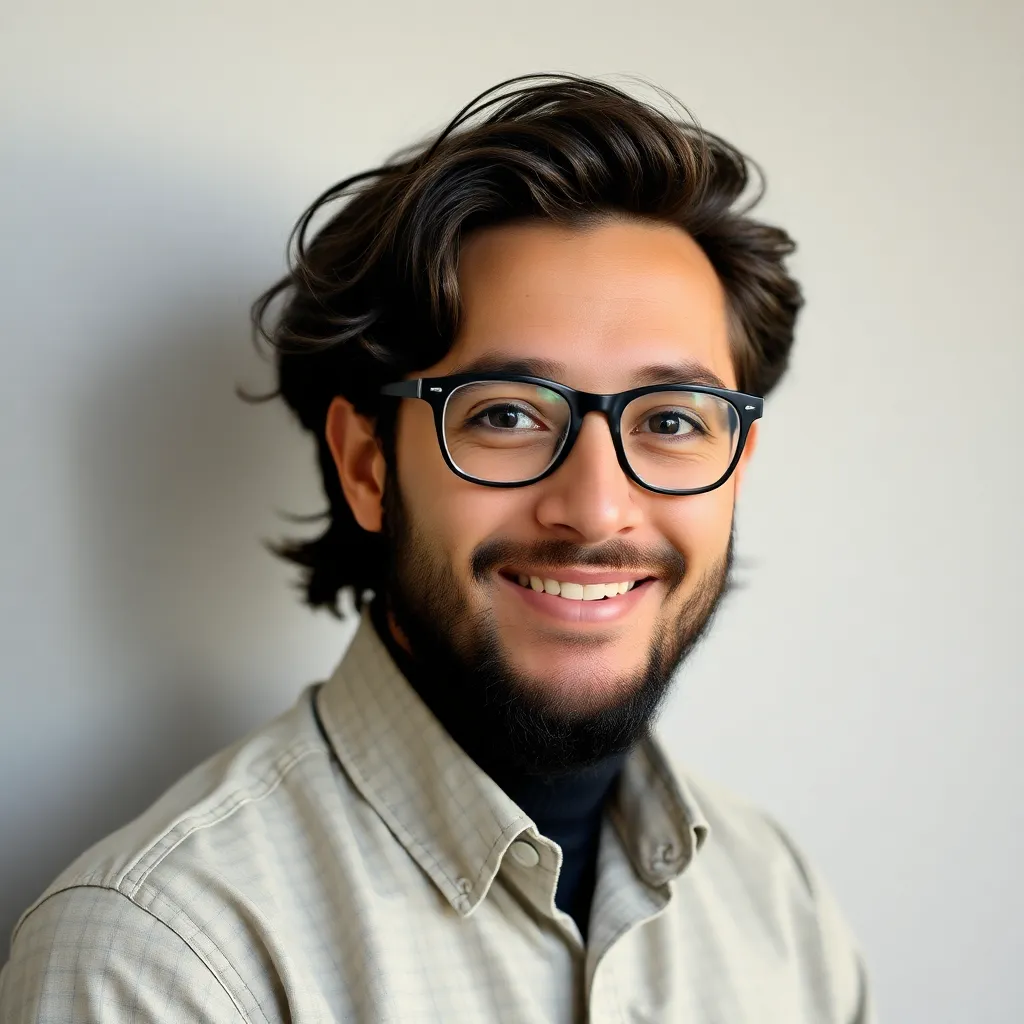
Muz Play
Apr 24, 2025 · 6 min read

Table of Contents
How to Find Energy Levels of an Element: A Comprehensive Guide
Determining the energy levels of an element is a fundamental concept in atomic physics and chemistry. Understanding these energy levels is crucial for predicting an element's chemical behavior, its spectral lines, and its interactions with electromagnetic radiation. This comprehensive guide will explore various methods and techniques used to determine the energy levels of elements, from basic theoretical models to advanced experimental techniques.
Understanding Atomic Energy Levels
Before diving into the methods, let's establish a foundational understanding. Electrons within an atom exist in specific energy levels, often visualized as shells or orbitals. These levels are quantized, meaning electrons can only occupy discrete energy states, not continuous values. The lowest energy level is called the ground state, while higher energy levels are called excited states. The energy difference between these levels dictates how an atom interacts with light and other forms of electromagnetic radiation.
The Bohr Model: A Simplified Approach
While a rudimentary model, the Bohr model provides a useful starting point. It postulates that electrons orbit the nucleus in specific, quantized orbits. The energy of each orbit is directly related to its distance from the nucleus; electrons in closer orbits have lower energy. This model successfully predicted the spectral lines of hydrogen, but it falls short for more complex atoms.
The Quantum Mechanical Model: A More Accurate Description
The quantum mechanical model provides a far more accurate description of atomic energy levels. It utilizes the Schrödinger equation, a complex mathematical equation that describes the behavior of electrons as wave functions. Solving this equation yields a set of wave functions, each representing a specific atomic orbital with a corresponding energy level. These orbitals are characterized by quantum numbers (principal quantum number, n; azimuthal quantum number, l; magnetic quantum number, ml; and spin quantum number, ms), which define the energy, shape, orientation, and spin of the electron within the orbital.
Key Concepts:
- Principal Quantum Number (n): Determines the energy level and size of the orbital (n = 1, 2, 3...). Higher n values correspond to higher energy levels and larger orbitals.
- Azimuthal Quantum Number (l): Determines the shape of the orbital (l = 0, 1, 2... n-1). l = 0 corresponds to an s orbital (spherical), l = 1 to a p orbital (dumbbell-shaped), l = 2 to a d orbital, and so on.
- Magnetic Quantum Number (ml): Determines the orientation of the orbital in space (ml = -l, -l+1... 0... l-1, l).
- Spin Quantum Number (ms): Represents the intrinsic angular momentum of the electron (+1/2 or -1/2).
Methods for Determining Energy Levels
Determining the precise energy levels of an element involves both theoretical calculations and experimental measurements. The complexity of the method depends on the element's atomic number and the desired level of accuracy.
1. Spectroscopic Methods: Observing the Light
Spectroscopy is arguably the most common and powerful technique for determining atomic energy levels. When an atom absorbs energy (e.g., from heating or an electrical discharge), its electrons jump to higher energy levels (excited states). When these electrons return to lower energy levels, they emit photons of light with energies corresponding to the energy difference between the levels. By analyzing the wavelengths (or frequencies) of emitted light, we can determine the energy differences and thus the energy levels themselves.
Types of Spectroscopy:
- Atomic Emission Spectroscopy (AES): Measures the wavelengths of light emitted by excited atoms.
- Atomic Absorption Spectroscopy (AAS): Measures the wavelengths of light absorbed by atoms in their ground state.
- UV-Vis Spectroscopy: Utilizes ultraviolet and visible light to study electronic transitions.
- X-ray Spectroscopy: Employs X-rays to probe inner-shell electron transitions.
Analyzing Spectral Data:
Spectral lines are represented as peaks in a spectrum. The position of each peak corresponds to a specific wavelength, which is related to the energy of the emitted or absorbed photon by the equation: E = hc/λ, where h is Planck's constant, c is the speed of light, and λ is the wavelength. By carefully analyzing the spectral lines, we can deduce the energy differences between the atomic energy levels. The Rydberg formula provides a useful tool for analyzing the hydrogen spectrum and other simple atomic spectra.
2. Photoelectron Spectroscopy (PES): Direct Measurement of Ionization Energies
Photoelectron spectroscopy offers a direct method for determining ionization energies, which are the energies required to remove an electron from an atom or molecule. In PES, a sample is irradiated with high-energy photons (e.g., X-rays or ultraviolet light). The energy of the emitted photoelectrons is then measured. The kinetic energy of the emitted electron is directly related to the binding energy (ionization energy) of the electron in the atom. The resulting spectrum shows peaks corresponding to different ionization energies, providing direct information about the energy levels of the atom.
3. Computational Methods: Theoretical Calculations
While experimental methods provide direct measurements, computational methods play a vital role, particularly for complex atoms. Sophisticated quantum mechanical calculations, using methods such as Hartree-Fock or Density Functional Theory (DFT), can predict the energy levels of atoms with high accuracy. These calculations involve solving the Schrödinger equation (or approximations thereof) for the atom, yielding a set of energy levels and corresponding wave functions. The accuracy of these calculations depends on the level of approximation used and the computational resources available.
4. Auger Electron Spectroscopy: Studying Core-Level Transitions
Auger electron spectroscopy is a surface-sensitive technique that probes the core-level energy levels of atoms. When a core-level electron is ejected (e.g., by X-ray irradiation), a higher-energy electron fills the vacancy. This transition releases energy, which can be transferred to another electron, causing its ejection (an Auger electron). The kinetic energy of the Auger electron is related to the energy levels of the core electrons, providing information about the core-level energy structure.
Factors Affecting Energy Levels
Several factors influence the energy levels of an atom:
- Nuclear Charge: A higher nuclear charge attracts electrons more strongly, resulting in lower energy levels.
- Shielding Effect: Inner electrons shield outer electrons from the full nuclear charge, reducing the effective nuclear charge experienced by outer electrons and increasing their energy levels.
- Electron-Electron Repulsion: Repulsion between electrons increases their energy levels.
- Relativistic Effects: At high atomic numbers, relativistic effects become significant, affecting the energy levels of inner-shell electrons.
Applications of Energy Level Determination
Understanding and determining the energy levels of elements has numerous applications in various scientific fields:
- Spectroscopy: Used for elemental analysis, material characterization, and environmental monitoring.
- Laser Technology: Precisely tuned lasers rely on understanding atomic energy levels for their operation.
- Astrophysics: Analyzing the spectra of stars and other celestial objects to determine their composition.
- Chemistry: Predicting the chemical reactivity and bonding properties of elements.
- Nuclear Physics: Studying nuclear transitions and decay processes.
- Materials Science: Designing new materials with specific electronic and optical properties.
Conclusion
Determining the energy levels of an element is a multifaceted process involving both experimental techniques and theoretical calculations. Spectroscopy, photoelectron spectroscopy, and computational methods provide valuable information about the energy levels of atoms, which is crucial for a wide range of scientific applications. The complexity of the method employed depends largely on the specific element and the desired level of accuracy. While the Bohr model offers a simplified understanding, the quantum mechanical model provides a far more accurate and comprehensive description of atomic energy levels. Continued research and advancements in experimental and computational techniques will undoubtedly further refine our understanding of this fundamental aspect of atomic structure.
Latest Posts
Latest Posts
-
What Is The Charge Of A Calcium Ion
Apr 24, 2025
-
Which Of The Following Are Characteristics Of Prokaryotes
Apr 24, 2025
-
The Measure Of Energy Of Motion Of Particles Of Matter
Apr 24, 2025
-
How Many Molecules Of Atp Were Produced During Cellular Respiration
Apr 24, 2025
-
Where Does The Citric Acid Cycle Occur In Eukaryotes
Apr 24, 2025
Related Post
Thank you for visiting our website which covers about How To Find Energy Levels Of An Element . We hope the information provided has been useful to you. Feel free to contact us if you have any questions or need further assistance. See you next time and don't miss to bookmark.