How To Get Ph From A E Cell Value
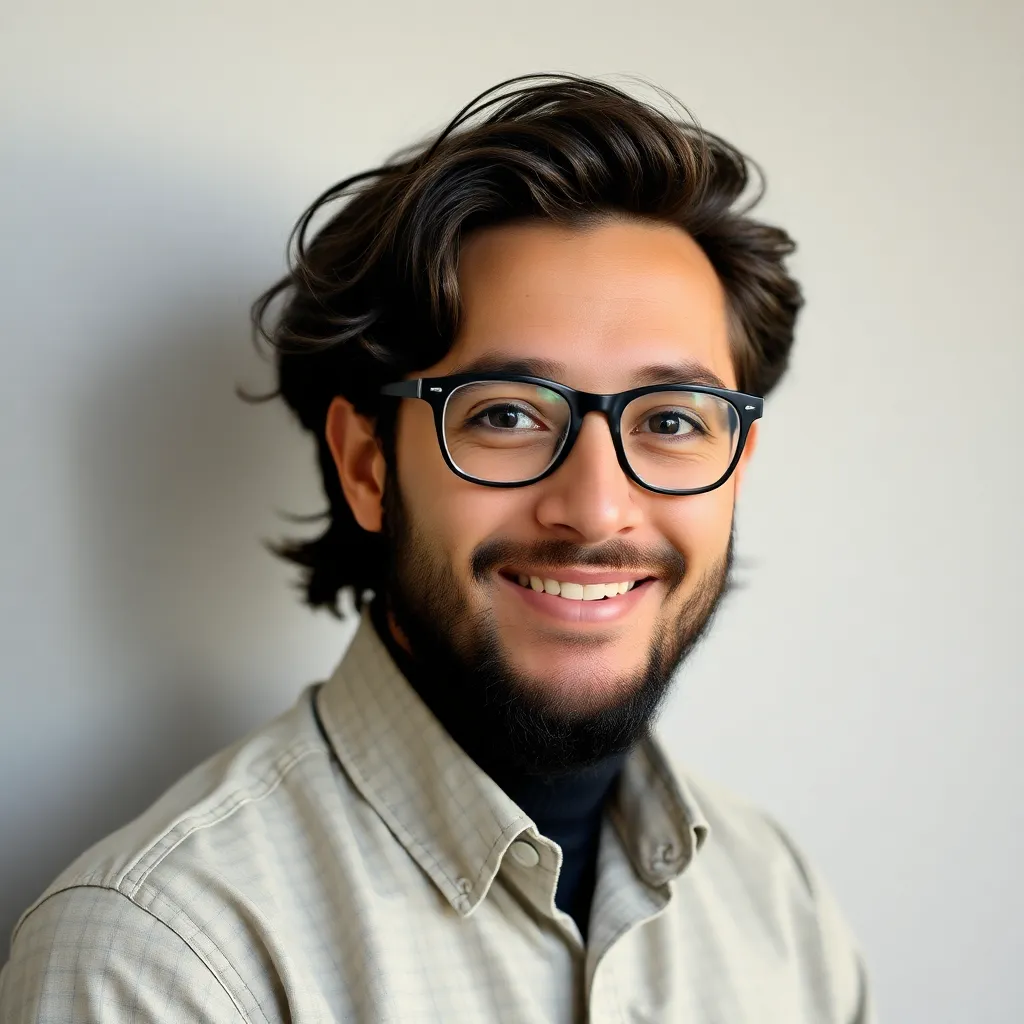
Muz Play
Mar 11, 2025 · 6 min read

Table of Contents
How to Get pH from an Electrochemical Cell (EC) Value: A Comprehensive Guide
Determining the pH of a solution is crucial across numerous scientific and industrial applications. While traditional methods like using pH indicators and pH meters offer straightforward solutions, understanding how pH relates to electrochemical cell (EC) measurements provides a powerful alternative, especially in automated systems or situations requiring continuous monitoring. This article delves into the intricacies of extracting pH information from electrochemical cell values, exploring the underlying principles, necessary calculations, and practical considerations.
Understanding the Fundamentals: pH and Electrochemical Cells
Before we delve into the specifics of extracting pH from EC values, let's establish a foundational understanding of both concepts.
What is pH?
pH, or potential of hydrogen, is a measure of the acidity or basicity (alkalinity) of a solution. It's defined as the negative logarithm (base 10) of the hydrogen ion concentration ([H⁺]) in moles per liter (M):
pH = -log₁₀[H⁺]
A pH of 7 indicates neutrality, values below 7 are acidic, and values above 7 are alkaline (basic). A change of one pH unit represents a tenfold change in hydrogen ion concentration.
Electrochemical Cells (EC) and their Relation to pH
Electrochemical cells, or galvanic cells, are devices that generate an electric potential (voltage) from a chemical reaction. For pH measurement, we're interested in specific types of electrochemical cells, often involving electrodes sensitive to hydrogen ion concentration. These cells typically employ:
-
A reference electrode: This electrode maintains a constant and known potential, independent of the solution's composition. Common examples include the saturated calomel electrode (SCE) and the silver/silver chloride electrode (Ag/AgCl).
-
A pH-sensitive electrode (indicator electrode): This electrode's potential varies proportionally with the hydrogen ion concentration of the solution. The most common type is the glass electrode, whose potential changes depending on the difference in hydrogen ion concentration across a thin glass membrane.
The overall cell potential (E<sub>cell</sub>) is the difference between the potentials of the reference and indicator electrodes. This potential is directly related to the pH of the solution.
The Nernst Equation: The Bridge Between EC Value and pH
The relationship between the cell potential (E<sub>cell</sub>) and pH is governed by the Nernst equation. This equation describes the potential of an electrochemical cell under non-standard conditions:
E<sub>cell</sub> = E⁰<sub>cell</sub> - (RT/nF) * ln(Q)
Where:
- E<sub>cell</sub> is the cell potential under the given conditions.
- E⁰<sub>cell</sub> is the standard cell potential (at standard conditions, usually 25°C and 1 M concentration).
- R is the ideal gas constant (8.314 J/mol·K).
- T is the temperature in Kelvin.
- n is the number of moles of electrons transferred in the cell reaction.
- F is the Faraday constant (96485 C/mol).
- Q is the reaction quotient, which depends on the concentrations of the species involved in the cell reaction.
For a pH-sensitive cell, the Nernst equation can be simplified and expressed in terms of pH:
E<sub>cell</sub> = E⁰<sub>cell</sub> + (2.303RT/nF) * pH
Simplified Nernst Equation for pH Measurement (at 25°C):
E<sub>cell</sub> ≈ E⁰<sub>cell</sub> + 0.0592 * pH (for n=1)
This simplified form shows a direct linear relationship between the cell potential (E<sub>cell</sub>) and pH at 25°C. The slope is approximately 0.0592 volts per pH unit.
Calibrating the Electrochemical Cell
Before using an electrochemical cell to measure pH, meticulous calibration is essential. This process involves measuring the cell potential (E<sub>cell</sub>) in solutions of known pH (buffer solutions). Typically, two or more buffer solutions with different pH values (e.g., pH 4.01, 7.00, and 10.01) are employed.
Calibration involves plotting the measured E<sub>cell</sub> values against the known pH values. This creates a calibration curve. The slope and intercept of the calibration curve are then used to calculate the pH of an unknown solution based on its measured E<sub>cell</sub> value.
Importance of Calibration:
- Accuracy: Calibration ensures accurate pH measurements by accounting for variations in electrode response and temperature.
- Compensation for Non-Idealities: The simplified Nernst equation makes certain assumptions. Calibration helps correct for deviations from ideal behavior.
- Maintaining Accuracy: Regular calibration is crucial to maintain the accuracy of the pH measurements over time.
Calculating pH from the Electrochemical Cell Value
Once the electrochemical cell is calibrated, calculating the pH from a measured E<sub>cell</sub> value involves using the equation derived from the calibration curve. This equation typically has the form:
pH = m * E<sub>cell</sub> + c
Where:
- m is the slope of the calibration curve.
- c is the y-intercept of the calibration curve.
Simply substitute the measured E<sub>cell</sub> value into this equation to obtain the pH of the unknown solution.
Practical Considerations and Potential Errors
While the process seems straightforward, several practical aspects need careful attention:
Temperature Effects
Temperature significantly influences the cell potential and the slope of the calibration curve. Therefore, temperature compensation is crucial for accurate pH measurement. Many pH meters incorporate automatic temperature compensation (ATC) or require manual temperature adjustment.
Electrode Maintenance and Fouling
Electrodes are sensitive and prone to fouling (coating with contaminants). Regular cleaning and storage are crucial. Fouling can lead to inaccurate measurements and requires proper cleaning procedures using appropriate solutions.
Junction Potential
The liquid junction potential, the potential difference at the interface between two solutions with different compositions, is another source of error. This effect can be minimized but not entirely eliminated through careful experimental design.
Electrode Drift
Over time, electrodes can drift in their response, leading to deviations from the calibration curve. Regular calibration helps mitigate this issue.
Advanced Techniques and Applications
Beyond the basic principles outlined, several advanced techniques enhance the accuracy and applicability of pH determination from electrochemical cell values.
ISFET Sensors
Ion-sensitive field-effect transistors (ISFETs) offer a miniaturized and integrated approach to pH sensing. They combine the advantages of electrochemical measurements with the compactness and ease of integration of semiconductor technology.
Microfluidic Systems
Integrating electrochemical cells within microfluidic systems allows for high-throughput, automated pH measurements in miniaturized environments. This is particularly useful in applications like drug discovery and environmental monitoring.
Continuous Monitoring
Continuous monitoring of pH is crucial in various processes, from fermentation to wastewater treatment. Electrochemical cells provide a convenient method for real-time, continuous pH measurement.
Conclusion
Determining pH from electrochemical cell (EC) values provides a robust and versatile method for pH measurement, especially in automated and continuous monitoring systems. While understanding the fundamental principles of electrochemistry and the Nernst equation is essential, accurate results demand careful calibration, proper electrode maintenance, and awareness of potential sources of error. By combining a strong theoretical foundation with meticulous experimental practice, researchers and engineers can effectively utilize electrochemical cells to gain precise and reliable pH information. The application of advanced techniques, such as ISFET sensors and microfluidic integration, further expands the capabilities and potential applications of this method.
Latest Posts
Latest Posts
-
The Average Kinetic Energy Of The Gas Molecules Is
May 09, 2025
-
Aldehydes And Ketones May Be Reduced To
May 09, 2025
-
Can Rate Constant K Be Negative
May 09, 2025
-
An Electrolyte Will Yield Ions By Undergoing A
May 09, 2025
-
Reactants Of The Electron Transport Chain
May 09, 2025
Related Post
Thank you for visiting our website which covers about How To Get Ph From A E Cell Value . We hope the information provided has been useful to you. Feel free to contact us if you have any questions or need further assistance. See you next time and don't miss to bookmark.