Identifying A Molecule From Its Electrostatic Potential Map
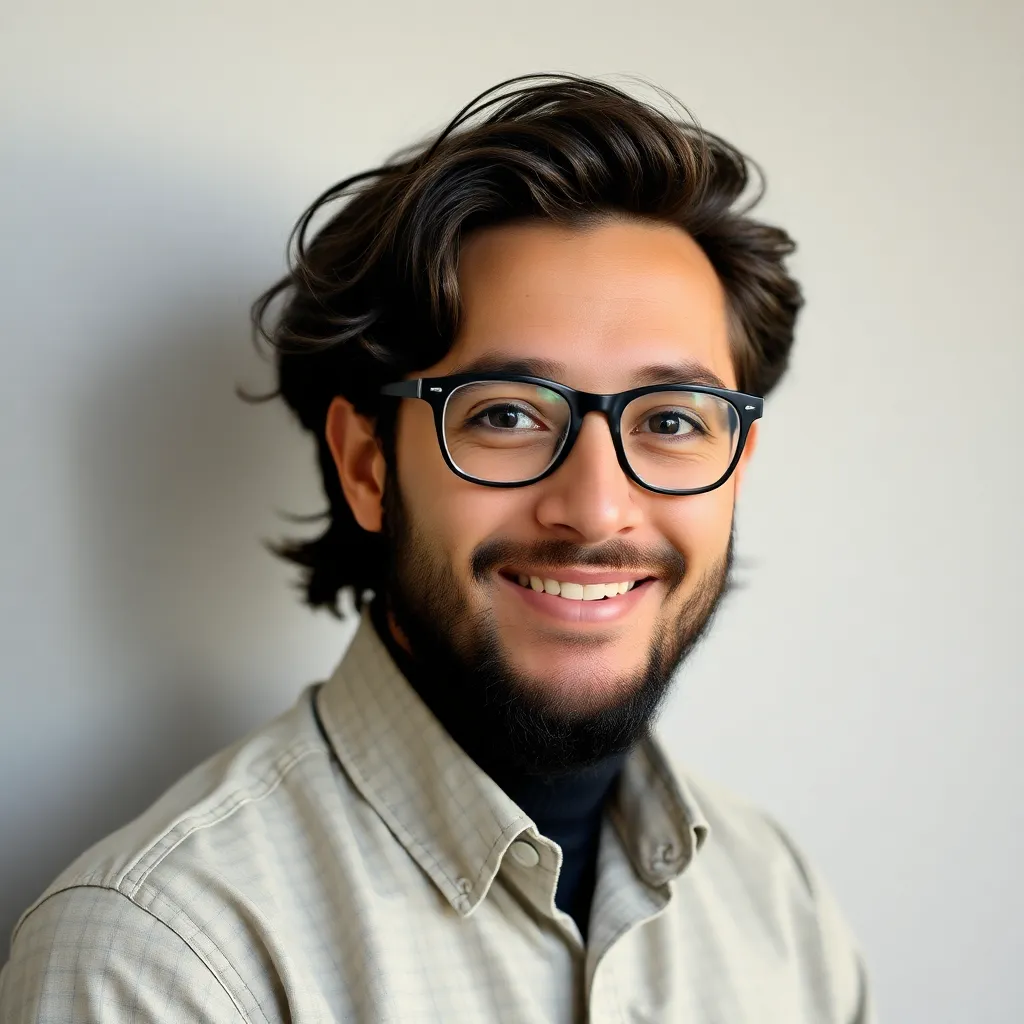
Muz Play
Apr 25, 2025 · 5 min read

Table of Contents
Identifying a Molecule from its Electrostatic Potential Map
Electrostatic potential (ESP) maps provide a powerful visual representation of the charge distribution within a molecule. Analyzing these maps is crucial in various fields, from drug discovery to materials science, allowing researchers to understand molecular reactivity, intermolecular interactions, and overall properties. This article delves deep into the process of identifying a molecule from its ESP map, exploring the underlying principles, practical techniques, and challenges involved.
Understanding Electrostatic Potential Maps
An ESP map depicts the electrostatic potential at various points surrounding a molecule. Regions of high electron density (e.g., lone pairs, negatively charged atoms) appear as red or negative potentials, while regions of low electron density (e.g., positively charged atoms, electron-deficient bonds) show up as blue or positive potentials. The color scale usually represents the magnitude of the electrostatic potential, with a smooth transition between colors indicating a gradual change in potential.
Key Features of an ESP Map:
-
Positive and Negative Regions: The presence and location of positively and negatively charged regions directly relate to the molecule's functional groups and overall polarity. A strongly polar molecule will exhibit distinct red and blue regions.
-
Magnitude of Potential: The intensity of the red and blue colors indicates the strength of the charge. Darker colors represent stronger charge concentrations.
-
Shape and Symmetry: The overall shape and symmetry of the ESP map provide clues about the molecular structure and symmetry elements. Symmetrical molecules will generally exhibit symmetrical ESP maps.
-
Isocontours: These lines connect points of equal electrostatic potential, further highlighting regions of similar charge distribution. Analyzing their arrangement can be vital for identifying molecular features.
Deciphering an ESP Map: A Step-by-Step Guide
Identifying a molecule solely from its ESP map can be challenging, particularly for complex molecules. However, a systematic approach combining visual inspection with computational tools can significantly enhance the identification process.
1. Visual Inspection:
-
Identifying Functional Groups: Look for characteristic patterns. For example, a concentrated red region might indicate an oxygen atom with lone pairs (like in a carbonyl group or alcohol), while a blue region might signify a positively charged hydrogen atom (like in an amine).
-
Assessing Molecular Polarity: Determine the overall polarity by observing the distribution of red and blue regions. A predominantly red map suggests a highly negative molecule, a predominantly blue map suggests a highly positive molecule, while a balanced distribution indicates a less polar molecule.
-
Recognizing Symmetry: Note any symmetry elements in the map (planes, axes, centers of symmetry). This can help narrow down the possible molecular structures significantly.
-
Estimating Molecular Size and Shape: The overall extent of the ESP map provides a rough estimate of the molecule's size. The shape gives clues about its overall geometry (linear, planar, tetrahedral, etc.).
2. Computational Tools and Techniques:
-
Molecular Modeling Software: Programs such as Gaussian, Spartan, and Avogadro allow for the generation and visualization of ESP maps. These programs also provide further computational analysis.
-
Database Searching: Several databases exist containing calculated ESP maps for a vast library of molecules. By comparing the unknown ESP map with those in the database, a potential match can be identified. Similarity measures can quantify the degree of similarity between maps.
-
Spectroscopic Data Correlation: If available, compare features of the ESP map with other spectroscopic data (e.g., NMR, IR, Mass spectrometry). Correlating these data points significantly strengthens the identification.
-
Quantum Chemical Calculations: Perform quantum mechanical calculations (e.g., DFT, HF) to determine the theoretical ESP map for candidate molecules. Compare this to the experimental ESP map for a definitive match. Energy minimization techniques further refine the structures for accurate comparisons.
3. Iterative Refinement:
Identifying a molecule from its ESP map is rarely a one-step process. It often involves an iterative process of refining hypotheses based on the visual inspection and computational analysis.
Challenges and Limitations:
While ESP maps offer valuable information, several challenges and limitations exist:
-
Resolution and Noise: Experimental ESP maps can be affected by noise and limited resolution, making it difficult to accurately interpret finer details. This is especially true for large or complex molecules.
-
Conformational Isomers: Many molecules exist in multiple conformations. Each conformation generates a unique ESP map. Distinguishing between them requires consideration of steric factors and conformational energy.
-
Ambiguity in Interpretation: Even with careful analysis, there might be ambiguities in interpreting ESP maps. Multiple molecules could potentially exhibit similar ESP patterns, especially for less polar or simpler molecules.
-
Computational Cost: Accurate quantum chemical calculations can be computationally demanding, especially for large molecules. This limits the feasibility of using high-level methods for rapid screening of numerous candidate molecules.
Advanced Techniques and Applications:
-
Mapping Reactive Sites: ESP maps pinpoint electrophilic and nucleophilic sites, crucial for predicting reaction pathways and designing new molecules with specific reactivity profiles.
-
Protein-Ligand Interactions: In drug discovery, ESP maps are instrumental in visualizing and understanding the electrostatic interactions between proteins and drug candidates. This is vital for designing drugs with high binding affinity and specificity.
-
Materials Science: Understanding the ESP distribution in materials is essential for designing new materials with desired electrical, optical, and mechanical properties.
-
Surface Science: ESP mapping techniques are also used to study the surface chemistry of materials. The surface charge distribution plays a critical role in adsorption, catalysis and other surface processes.
Conclusion:
Identifying a molecule solely from its electrostatic potential map is a challenging yet rewarding task. A thorough understanding of the principles behind ESP maps combined with the strategic application of computational tools and a systematic approach leads to successful molecule identification. The iterative nature of this process often requires combining data from various sources and refining initial hypotheses until a conclusive match is established. While limitations and ambiguities can exist, the overall power and utility of ESP maps in various scientific disciplines are unquestionable. This method continues to evolve with advancements in computational capabilities and data analysis techniques, promising even more accurate and efficient molecular identification in the future.
Latest Posts
Latest Posts
-
Match The Name Of The Eukaryotic Organism With Its Description
Apr 25, 2025
-
11 1 Solving Linear Systems By Graphing
Apr 25, 2025
-
Central Chemoreceptors Located In The Medulla Provide Feedback
Apr 25, 2025
-
Who Said All Cells Come From Preexisting Cells
Apr 25, 2025
-
Which Atomic Particle Has No Charge
Apr 25, 2025
Related Post
Thank you for visiting our website which covers about Identifying A Molecule From Its Electrostatic Potential Map . We hope the information provided has been useful to you. Feel free to contact us if you have any questions or need further assistance. See you next time and don't miss to bookmark.