Identifying Intermediates In A Reaction Mechanism
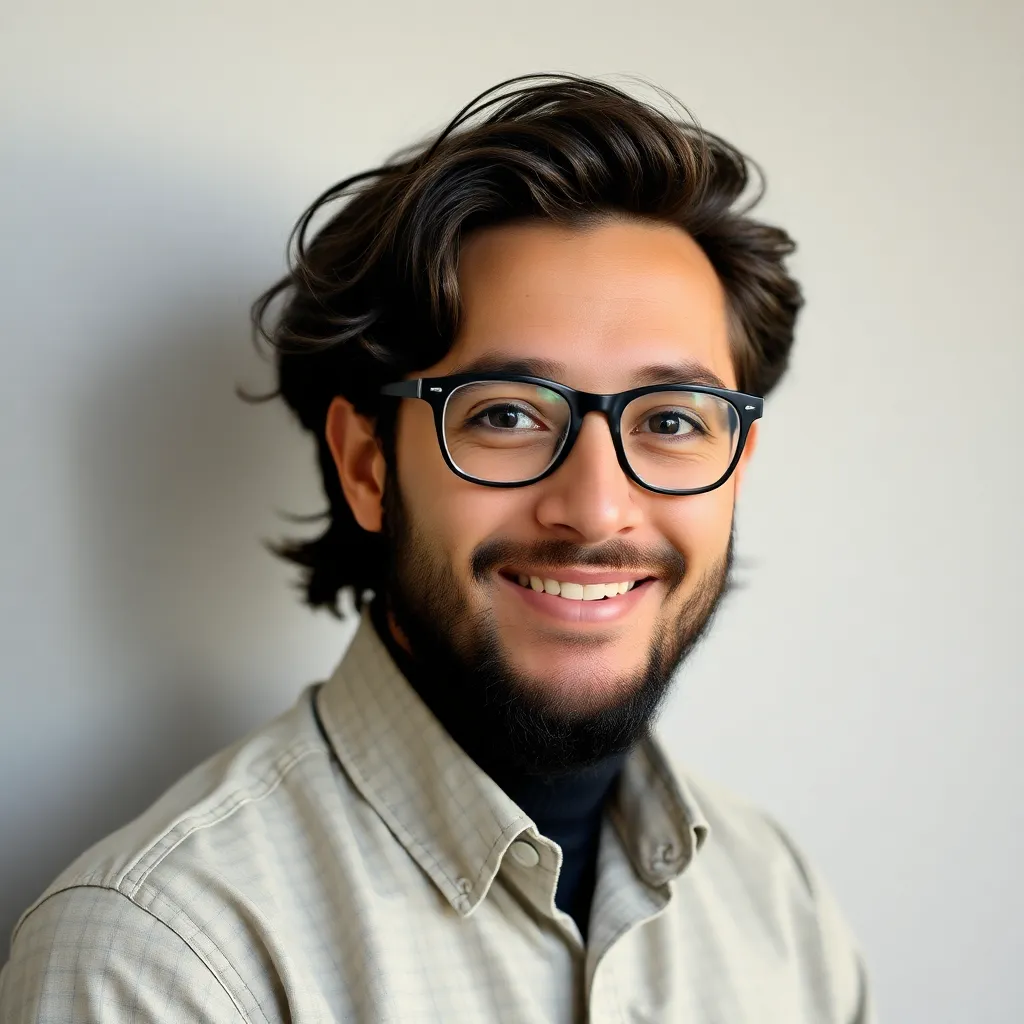
Muz Play
Apr 05, 2025 · 6 min read
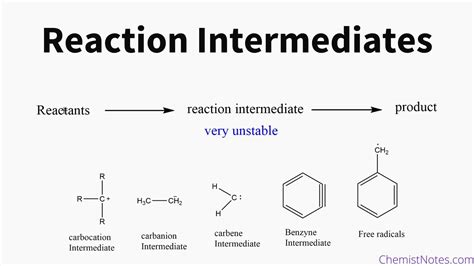
Table of Contents
Identifying Intermediates in a Reaction Mechanism: A Comprehensive Guide
Determining the precise mechanism of a chemical reaction is a cornerstone of chemistry. Understanding the step-by-step process not only explains how reactants transform into products but also allows for predictions about reaction rates, yields, and the influence of reaction conditions. A crucial aspect of elucidating reaction mechanisms is the identification of intermediates—short-lived, high-energy species that are neither reactants nor products. This article will delve into various techniques and strategies employed to identify these elusive intermediates.
Understanding Reaction Mechanisms and Intermediates
A reaction mechanism details the individual elementary steps involved in the overall transformation of reactants into products. Each elementary step represents a single molecular event, such as bond breaking, bond formation, or electron transfer. Intermediates are formed in one elementary step and consumed in a subsequent step, meaning they don't appear in the overall stoichiometric equation. Their transient nature makes their detection challenging.
Key Characteristics of Intermediates:
- High Energy: Intermediates typically possess higher energy than both reactants and products, existing in a local energy minimum along the reaction coordinate.
- Short Lifetime: Their high energy renders them highly reactive, resulting in short lifetimes.
- Not Present in Overall Stoichiometry: Intermediates are consumed before the reaction's completion and are therefore absent from the balanced chemical equation.
- Often Unstable: Many intermediates are highly reactive and unstable under normal conditions, further complicating their identification.
Experimental Techniques for Identifying Intermediates
Several sophisticated experimental techniques have been developed to identify and characterize intermediates. These techniques often rely on trapping or observing the intermediates under specific conditions to slow down their rapid reactions.
1. Spectroscopic Techniques: Capturing a Glimpse
Spectroscopic methods offer powerful tools for identifying intermediates. Different spectroscopic techniques exploit the interaction of the intermediate with electromagnetic radiation, providing information about its structure, energy levels, and reactivity.
- Infrared (IR) Spectroscopy: IR spectroscopy can identify functional groups present in the intermediate by detecting characteristic vibrational frequencies. This technique is particularly useful when dealing with relatively stable intermediates.
- Ultraviolet-Visible (UV-Vis) Spectroscopy: UV-Vis spectroscopy monitors the absorption of ultraviolet and visible light. The characteristic absorption wavelengths can be used to identify intermediates with chromophores—functional groups that absorb light in the UV-Vis region.
- Nuclear Magnetic Resonance (NMR) Spectroscopy: NMR spectroscopy provides detailed information about the structure and bonding within a molecule. While challenging for highly reactive intermediates due to their short lifetimes, advancements in techniques like low-temperature NMR and rapid-scan NMR are making it increasingly feasible.
- Electron Spin Resonance (ESR) Spectroscopy (also known as Electron Paramagnetic Resonance, EPR): ESR spectroscopy is highly sensitive to unpaired electrons. It's particularly useful for detecting radical intermediates, which possess one or more unpaired electrons. ESR allows for the detailed characterization of radical structures and their reactivity.
- Mass Spectrometry (MS): Mass spectrometry identifies intermediates based on their mass-to-charge ratio. This technique is particularly useful when coupled with other techniques like gas chromatography (GC-MS) or liquid chromatography (LC-MS). By separating the mixture components before detection, it's possible to isolate and analyze intermediates with greater ease.
2. Kinetic Studies: Unveiling Reaction Rates
Kinetic studies provide indirect evidence for intermediates by analyzing the rate law of the overall reaction. Deviations from simple rate laws often suggest the involvement of intermediates. Techniques like stopped-flow spectroscopy allow for rapid mixing of reactants and subsequent monitoring of the reaction progress, providing valuable insights into the formation and consumption of intermediates.
Analyzing Kinetic Isotope Effects (KIEs): Replacing an atom in a reactant with its heavier isotope can significantly alter reaction rates. The magnitude of this isotope effect provides crucial clues about the involvement of that atom in the rate-determining step and the nature of intermediates.
3. Trapping Experiments: Stabilizing the Elusive
Trapping experiments involve introducing a reagent specifically designed to react with the intermediate, forming a more stable adduct that can be isolated and characterized. This "trapping agent" effectively stabilizes the intermediate, preventing its rapid decay and allowing for its analysis.
4. Computational Chemistry: Predictive Modeling
Computational chemistry offers a powerful tool for predicting the existence and properties of intermediates. Quantum mechanical calculations allow the prediction of energy profiles, geometries, and vibrational frequencies of various possible intermediates. Comparing these predictions with experimental observations strengthens the evidence for proposed mechanisms.
Strategies for Identifying Intermediates
Beyond specific experimental techniques, several strategic approaches enhance the chances of successful intermediate identification.
1. Analyzing Reaction Conditions:
- Temperature: Lowering the reaction temperature can slow down the reaction, allowing for a longer lifetime for intermediates and easier detection.
- Solvent: Choosing an appropriate solvent can influence the stability and lifetime of intermediates.
- Concentration: Modifying reactant concentrations can help reveal the order of reactions and suggest the presence of intermediates.
2. Using Analogous Reactions:
Studying similar reactions with known mechanisms can offer valuable insights. If a similar reaction has a well-established mechanism involving a specific intermediate, it may suggest the presence of an analogous intermediate in the reaction under investigation.
3. Considering Stereochemistry:
Stereochemical outcomes can provide evidence for intermediate involvement. If the stereochemistry of the product is inconsistent with a direct transformation from reactants, it suggests the participation of an intermediate.
4. Combining Experimental and Computational Approaches:
Combining experimental techniques with computational methods is a powerful synergy. Computational predictions can guide experimental design and analysis, while experimental data validates or refines computational models.
Examples of Intermediate Identification in Specific Reactions
Several classic examples illustrate the application of these techniques.
1. SN1 Reactions: In SN1 (substitution nucleophilic unimolecular) reactions, a carbocation intermediate is formed. Its existence can be supported by spectroscopic evidence and kinetic studies demonstrating a first-order rate dependence on the substrate.
2. SN2 Reactions: While SN2 reactions proceed via a concerted mechanism, without distinct intermediates, careful kinetic isotope studies can reveal details of the transition state, providing insights into the reaction mechanism.
3. Free Radical Reactions: Free radical intermediates are commonly identified using ESR spectroscopy, which detects the characteristic signal of unpaired electrons. Trapping experiments using radical scavengers provide further evidence.
4. Enzyme Catalysis: The study of enzyme mechanisms often relies on various spectroscopic techniques, site-directed mutagenesis, and sophisticated kinetic studies to identify short-lived enzyme-substrate intermediates.
Challenges and Limitations
Despite the advancements in experimental and computational techniques, several challenges remain in identifying intermediates:
- High Reactivity: The high reactivity and short lifetime of many intermediates make their detection difficult.
- Low Concentrations: The transient nature of intermediates often results in low concentrations, making their detection challenging.
- Complex Reaction Mechanisms: In reactions with multiple steps and multiple intermediates, untangling the details of the mechanism can be complex.
- Computational Limitations: While computational chemistry is a valuable tool, it relies on approximations and model assumptions, limiting the accuracy of predictions.
Conclusion
Identifying intermediates in a reaction mechanism is a challenging but crucial aspect of understanding chemical reactivity. A combination of experimental techniques, strategic approaches, and computational methods is often necessary to achieve this goal. The ongoing development of new techniques and the increasing power of computational chemistry continue to enhance our ability to unveil the intricate details of even the most complex reaction mechanisms. The successful identification of intermediates leads to a more profound understanding of reaction pathways, improving our ability to design and optimize chemical processes. This knowledge extends across diverse fields, from organic chemistry and materials science to biochemistry and drug development.
Latest Posts
Latest Posts
-
Polynomial Addition Subtraction And Multiplication Worksheet
Apr 05, 2025
-
Single Celled Organisms That Lack A Nucleus Ar
Apr 05, 2025
-
Shaft Of A Long Bone Is Called
Apr 05, 2025
-
The Three Statements Of The Cell Theory
Apr 05, 2025
-
What Characteristics Are Shared By All Living Things
Apr 05, 2025
Related Post
Thank you for visiting our website which covers about Identifying Intermediates In A Reaction Mechanism . We hope the information provided has been useful to you. Feel free to contact us if you have any questions or need further assistance. See you next time and don't miss to bookmark.