In Eukaryotic Cells Dna Has The Appearance Of A
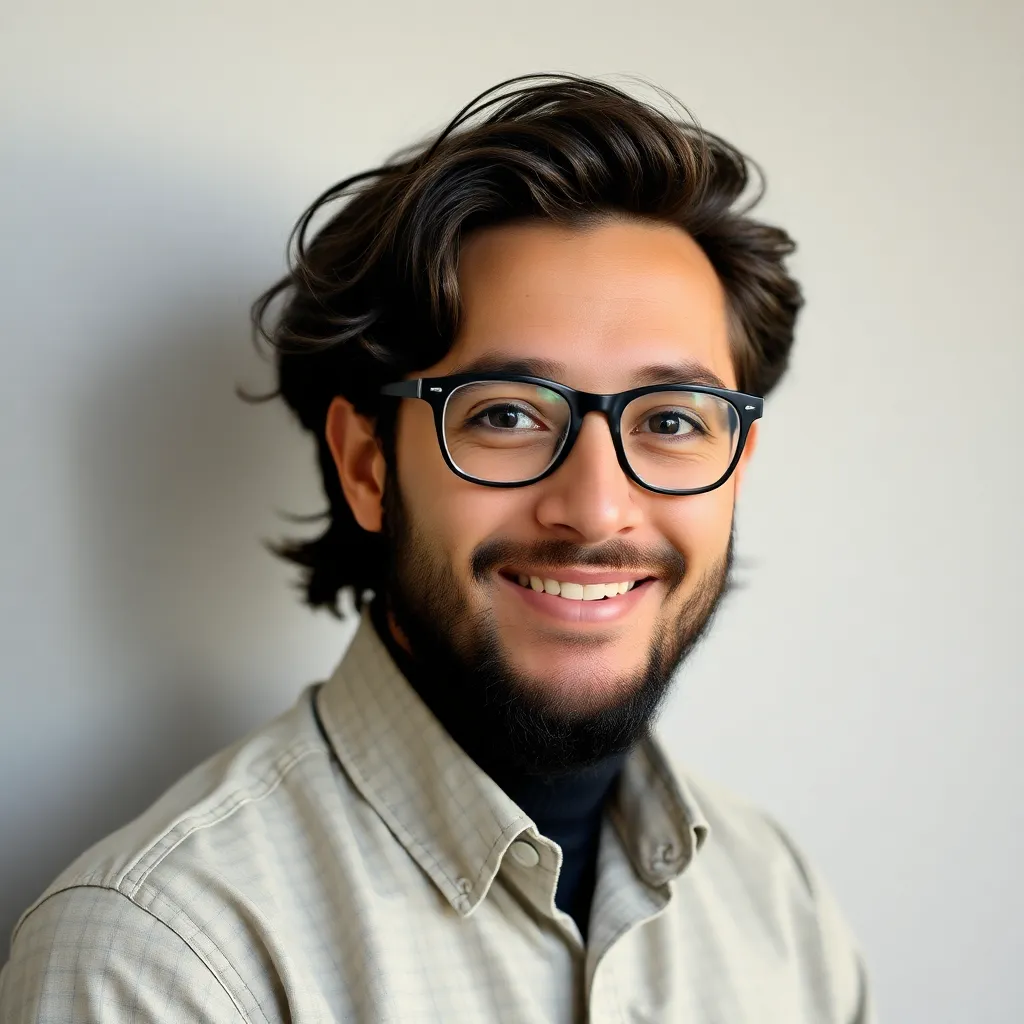
Muz Play
Apr 12, 2025 · 6 min read

Table of Contents
In Eukaryotic Cells, DNA Has the Appearance of a…Chromatin Fiber! Understanding Its Structure and Function
In eukaryotic cells, the genetic material, DNA, doesn't float freely within the nucleus. Instead, it's meticulously organized into a complex structure known as chromatin. This isn't simply a haphazard jumble; the appearance of DNA in eukaryotic cells is that of a highly organized and dynamic chromatin fiber, crucial for various cellular processes. Understanding this structure is key to grasping the intricacies of gene regulation, cell division, and overall genome stability.
The Fundamental Unit: The Nucleosome
The fundamental building block of chromatin is the nucleosome. Imagine a spool of thread; the thread represents the DNA double helix, and the spool is an octamer of histone proteins. These histones – specifically H2A, H2B, H3, and H4 – are highly conserved proteins that act as structural scaffolds around which the DNA wraps approximately 1.67 times. This wrapping compacts the DNA significantly, reducing its overall length.
Histone Tails: The Dynamic Regulators
The histone proteins possess "tails," N-terminal extensions that protrude from the nucleosome core. These tails are not static; they're subject to various post-translational modifications (PTMs). These modifications, including acetylation, methylation, phosphorylation, and ubiquitination, act as a sort of "histone code," influencing chromatin structure and gene expression. Acetylation, for instance, generally loosens chromatin structure, making DNA more accessible to transcription factors and promoting gene transcription. Conversely, methylation can have diverse effects, sometimes promoting gene activation and other times silencing it, depending on the specific residue and the number of methyl groups added.
From Nucleosomes to Chromatin Fibers: Levels of Organization
The nucleosomes themselves are not randomly arranged; they are further organized into higher-order structures. The arrangement of nucleosomes forms a 10-nm fiber, a string of beads-on-a-string appearance visible under electron microscopy. This is the most basic level of chromatin packaging beyond the nucleosome.
However, the 10-nm fiber is further compacted. Through interactions between linker histones (like histone H1) and nucleosomes, the 10-nm fiber folds into a 30-nm fiber, a more compact structure with a solenoid or zig-zag arrangement. The exact structure of the 30-nm fiber remains a subject of ongoing research, with different models proposed based on experimental data.
The 30-nm fiber is then organized into even higher-order structures, forming loops, rosettes, and ultimately chromosomes. These higher-order structures are crucial for efficiently packaging the vast amount of DNA within the nucleus. A human cell, for example, contains approximately 2 meters of DNA, yet the nucleus itself is only a few micrometers in diameter. This remarkable compaction is achieved through the hierarchical organization of chromatin.
Chromatin Remodeling: Dynamic Regulation of Gene Expression
Chromatin is not a static structure; its organization is constantly changing in response to cellular signals. Chromatin remodeling complexes, large multi-protein machines, actively alter chromatin structure. These complexes utilize ATP hydrolysis to reposition or evict nucleosomes, making DNA regions more or less accessible to transcription machinery.
This dynamic remodeling plays a pivotal role in regulating gene expression. When a gene needs to be expressed, chromatin remodeling complexes can loosen the chromatin structure in the vicinity of the gene's promoter, allowing transcription factors and RNA polymerase to bind and initiate transcription. Conversely, when a gene needs to be silenced, the chromatin can be compacted, making the DNA inaccessible and preventing transcription.
The Role of Epigenetics
The modifications on histone tails and the changes in chromatin structure are examples of epigenetic modifications. These are heritable changes in gene expression that do not involve alterations to the underlying DNA sequence. Epigenetic modifications are crucial for cellular differentiation, development, and responses to environmental stimuli. They influence which genes are active or inactive in different cell types, contributing to the diversity of cell functions within an organism.
Chromatin and Cell Division: Condensation and Chromosome Formation
During cell division (mitosis and meiosis), chromatin undergoes a dramatic transformation. The chromatin fibers condense extensively, forming the characteristic condensed chromosomes visible under a light microscope. This highly condensed state ensures accurate segregation of chromosomes during cell division, preventing DNA breakage and ensuring each daughter cell receives a complete copy of the genome.
The process of chromosome condensation involves further compaction of the chromatin fibers, involving additional proteins and interactions beyond the histone octamers and the 30-nm fiber. This extreme condensation ensures that the chromosomes are robust enough to withstand the mechanical stresses of cell division.
Euchromatin vs. Heterochromatin: A Tale of Two Chromatin States
Chromatin exists in two major states: euchromatin and heterochromatin. Euchromatin is a less condensed form of chromatin, generally associated with actively transcribed genes. It is more accessible to transcription factors and RNA polymerase, allowing for efficient gene expression.
In contrast, heterochromatin is a highly condensed form of chromatin, typically associated with silenced genes. It is largely inaccessible to the transcription machinery, effectively preventing gene expression. Heterochromatin often contains repetitive DNA sequences and is located at the periphery of the nucleus. There are two main types of heterochromatin: constitutive heterochromatin, which is always condensed, and facultative heterochromatin, which can switch between condensed and decondensed states depending on cellular needs.
The Importance of Chromatin Structure in Disease
Disruptions in chromatin structure and function are implicated in a wide range of human diseases, including cancer, developmental disorders, and neurological conditions. Mutations in histone proteins or chromatin remodeling complexes can lead to aberrant gene expression, contributing to disease pathogenesis. For instance, altered histone modifications are frequently observed in cancer cells, contributing to uncontrolled cell growth and proliferation. Understanding the intricacies of chromatin structure and its regulation is therefore crucial for developing effective diagnostic tools and therapeutic interventions for these diseases.
Future Directions: Unraveling the Mysteries of Chromatin
Despite significant advances in our understanding of chromatin, many mysteries remain. The precise three-dimensional organization of chromatin within the nucleus, the mechanisms governing chromatin remodeling dynamics, and the full extent of epigenetic regulation continue to be areas of active research. Technological advancements, such as advanced microscopy techniques and high-throughput sequencing, are providing new insights into these complex processes.
Conclusion: A Dynamic and Essential Structure
In eukaryotic cells, DNA's appearance is anything but random. It is meticulously organized into a dynamic chromatin fiber, a structure that elegantly balances compaction with accessibility. The intricate interplay between histone proteins, chromatin remodeling complexes, and epigenetic modifications ensures precise regulation of gene expression, genome stability, and successful cell division. Understanding the architecture and function of chromatin is paramount for deciphering the complexities of cellular life and developing treatments for a wide range of human diseases. The field continues to evolve, promising exciting breakthroughs in our understanding of this fundamental aspect of eukaryotic biology. Future research will undoubtedly further refine our understanding of this fascinating and vital structure.
Latest Posts
Latest Posts
-
Which Element Has 2 Valence Electrons
Apr 18, 2025
-
What Does The Upper Left Number In The Symbol Represent
Apr 18, 2025
-
What Energy Is Transferred By Electromagnetic Waves
Apr 18, 2025
-
The Diagram Above Represents A Typical Rod Shaped Bacterium
Apr 18, 2025
-
What Are Three Subatomic Particles In An Atom
Apr 18, 2025
Related Post
Thank you for visiting our website which covers about In Eukaryotic Cells Dna Has The Appearance Of A . We hope the information provided has been useful to you. Feel free to contact us if you have any questions or need further assistance. See you next time and don't miss to bookmark.