In Order For A Process To Be Spontaneous
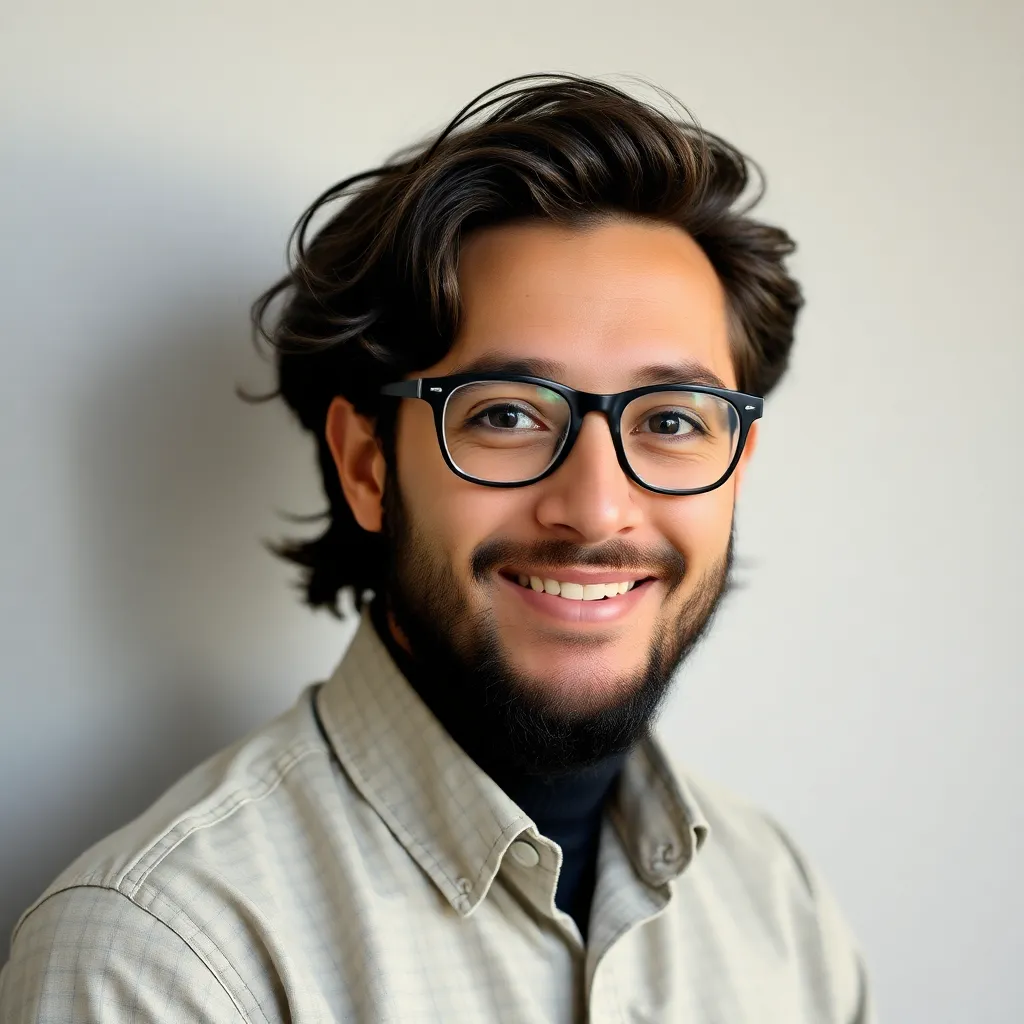
Muz Play
Mar 27, 2025 · 6 min read
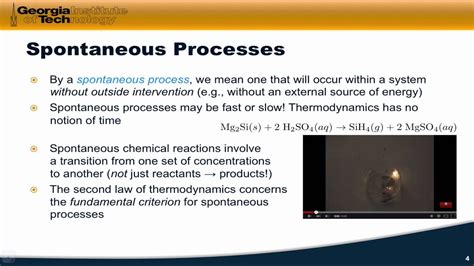
Table of Contents
In Order for a Process to be Spontaneous: A Deep Dive into Thermodynamics
Spontaneity, in the context of thermodynamics, doesn't refer to how quickly a process occurs, but rather whether it will occur at all without external intervention. A spontaneous process is one that proceeds in a particular direction under a given set of conditions without any continuous external influence. Understanding the conditions that dictate spontaneity is crucial in various fields, from chemistry and physics to materials science and biology. This article will delve into the intricate details of spontaneity, exploring the key thermodynamic functions that govern it.
The Role of Entropy in Spontaneous Processes
The second law of thermodynamics provides the fundamental principle for determining spontaneity: the total entropy of an isolated system can only increase over time or remain constant in ideal cases where the system is in a steady state or undergoing a reversible process. Entropy (S), often described as a measure of disorder or randomness, plays a central role. An increase in entropy signifies a greater dispersal of energy and matter within a system.
Understanding Entropy Change (ΔS)
The change in entropy (ΔS) for a process is given by:
ΔS = S<sub>final</sub> - S<sub>initial</sub>
A positive ΔS (ΔS > 0) indicates an increase in entropy, favoring spontaneity. A negative ΔS (ΔS < 0) signifies a decrease in entropy, making the process non-spontaneous under the given conditions. A ΔS of zero (ΔS = 0) suggests a reversible process where entropy remains constant.
Examples of Entropy Changes
Consider the following examples:
-
Melting of ice: The transition from a highly ordered solid (ice) to a less ordered liquid (water) involves an increase in entropy (ΔS > 0). This is a spontaneous process at temperatures above 0°C.
-
Expansion of a gas: When a gas expands into a vacuum, its molecules occupy a larger volume, resulting in increased randomness and higher entropy (ΔS > 0). This is a spontaneous process.
-
Formation of a crystal: The formation of a well-ordered crystal from a disordered liquid or gas involves a decrease in entropy (ΔS < 0). This process is generally non-spontaneous unless other factors, such as enthalpy changes, are favorable.
Enthalpy and Gibbs Free Energy: Further Determinants of Spontaneity
While entropy change provides valuable insight, it's not the sole determinant of spontaneity. The enthalpy (H), representing the total heat content of a system, also plays a significant role. Enthalpy changes (ΔH) reflect the heat absorbed or released during a process. Exothermic processes (ΔH < 0), releasing heat, tend to be more favorable, while endothermic processes (ΔH > 0), absorbing heat, are less so.
To combine the effects of enthalpy and entropy, we introduce the Gibbs free energy (G):
G = H - TS
where T is the absolute temperature. The change in Gibbs free energy (ΔG) for a process is:
ΔG = ΔH - TΔS
This equation is central to predicting spontaneity.
Spontaneity based on ΔG:
- ΔG < 0: The process is spontaneous under the given conditions.
- ΔG > 0: The process is non-spontaneous under the given conditions. The reverse process would be spontaneous.
- ΔG = 0: The process is at equilibrium. There is no net change in the forward or reverse direction.
The sign of ΔG depends on both ΔH and TΔS. At constant temperature and pressure, a negative ΔG is the criterion for spontaneity.
Temperature's Influence on Spontaneity
The temperature's effect on spontaneity is crucial because it's a multiplier for the entropy term (TΔS) in the Gibbs free energy equation. This means that even if a process has a positive ΔH (endothermic), it can still be spontaneous if the TΔS term is sufficiently large and positive.
-
High Temperatures: At high temperatures, the TΔS term dominates. Even endothermic processes with a positive ΔH can become spontaneous if the entropy increase (ΔS > 0) is large enough. For example, the melting of ice is endothermic but spontaneous at temperatures above 0°C because the entropy increase outweighs the enthalpy increase.
-
Low Temperatures: At low temperatures, the TΔS term is small. Spontaneity is primarily determined by the enthalpy change (ΔH). Exothermic processes (ΔH < 0) are generally favored at low temperatures.
Non-Standard Conditions: The Influence of Concentration and Pressure
The Gibbs free energy equation discussed above applies to standard conditions (typically 298 K and 1 atm). However, in real-world scenarios, conditions often deviate from standard values. The impact of concentration and pressure on spontaneity can be incorporated using the following equation:
ΔG = ΔG° + RTlnQ
Where:
- ΔG°: Standard Gibbs free energy change
- R: Ideal gas constant
- T: Temperature (in Kelvin)
- Q: Reaction quotient (a measure of the relative amounts of reactants and products at any given point)
The reaction quotient (Q) accounts for deviations from standard states. For example, in chemical reactions, the concentrations of reactants and products significantly influence the spontaneity. A high concentration of reactants will favor the forward reaction (product formation), and vice versa. Similarly, in gas-phase reactions, changes in pressure can influence the equilibrium position and spontaneity.
Applications of Spontaneity Principles
The principles of spontaneity have far-reaching applications across many scientific and engineering disciplines:
-
Chemistry: Predicting the feasibility of chemical reactions, understanding equilibrium constants, and designing efficient synthesis routes.
-
Materials Science: Designing materials with desired properties by controlling the spontaneity of phase transitions and other processes. Understanding the stability of materials and their resistance to degradation.
-
Biology: Exploring metabolic processes, understanding the driving forces behind biological reactions, and analyzing energy transformations in living systems. For example, understanding why certain biochemical reactions are spontaneous within a cell while others require enzyme catalysis.
-
Environmental Science: Analyzing environmental processes like pollutant degradation or the spontaneous formation of certain compounds in the atmosphere. Understanding the driving forces behind these processes is crucial for environmental management.
Limitations and Considerations
While the concepts of entropy, enthalpy, and Gibbs free energy are powerful tools for predicting spontaneity, certain limitations must be acknowledged:
-
Reaction Kinetics: Spontaneity does not dictate the rate of a reaction. A spontaneous reaction can be extremely slow if the activation energy is high. Kinetics provides insight into the reaction speed, which is a separate consideration from spontaneity.
-
Equilibrium vs. Spontaneity: Spontaneity refers to the direction of a process under given conditions, while equilibrium describes the state where the forward and reverse rates are equal. A spontaneous process might reach equilibrium only after progressing to a certain extent.
-
Isolated vs. Open/Closed Systems: The second law of thermodynamics, which underpins spontaneity, is strictly applicable to isolated systems. Open and closed systems exchange energy or matter with their surroundings, and the analysis of spontaneity becomes more complex.
Conclusion
The spontaneity of a process is a critical concept in thermodynamics. The interplay of entropy and enthalpy, quantified through the Gibbs free energy, determines whether a process will proceed without continuous external influence. Understanding the effects of temperature, concentration, and pressure on spontaneity is crucial for various applications in diverse fields. While the Gibbs free energy provides a powerful framework, it's crucial to remember the limitations and to consider other factors, such as reaction kinetics and the nature of the system, for a complete understanding of thermodynamic processes. The principles of spontaneity are foundational to many scientific advancements and technological innovations, offering a deep understanding of the natural world and our ability to harness its processes.
Latest Posts
Latest Posts
-
How To Test Symmetry Of Pole
Mar 30, 2025
-
Identification Of Unknown Bacteria Lab Report Pdf
Mar 30, 2025
-
Difference Between The Autonomic And Somatic Nervous System
Mar 30, 2025
-
Examples Of Literary Analysis Thesis Statements
Mar 30, 2025
-
Why Might Two Elements Possess Similar Chemical Properties
Mar 30, 2025
Related Post
Thank you for visiting our website which covers about In Order For A Process To Be Spontaneous . We hope the information provided has been useful to you. Feel free to contact us if you have any questions or need further assistance. See you next time and don't miss to bookmark.