In Prokaryotic Transcription How Is The Holoenzyme Rna Polymerase Formed
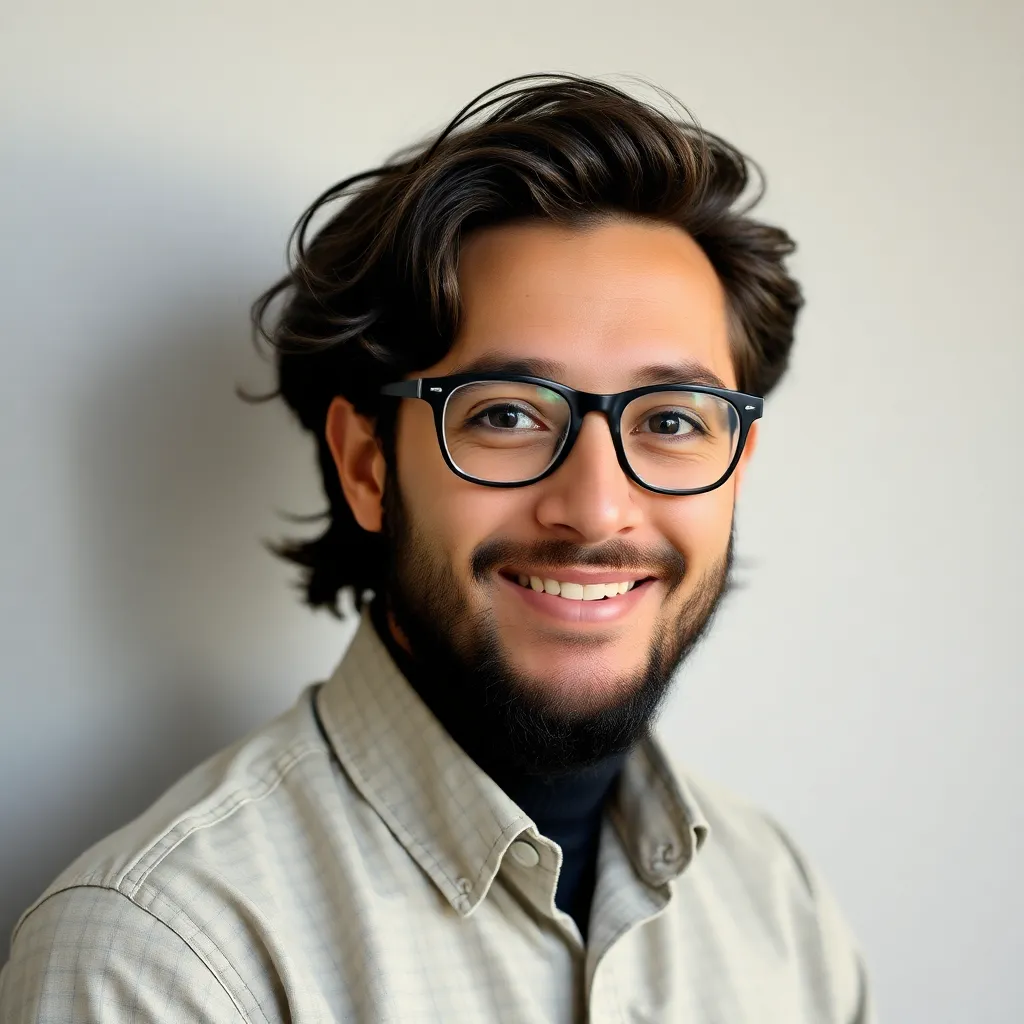
Muz Play
Apr 26, 2025 · 8 min read

Table of Contents
In Prokaryotic Transcription: How the Holoenzyme RNA Polymerase is Formed
Prokaryotic transcription, the process of synthesizing RNA from a DNA template, is a fundamental process in the life of bacteria and archaea. This process is orchestrated primarily by RNA polymerase, a complex enzyme that plays a crucial role in gene expression. Understanding how this enzyme, specifically its holoenzyme form, is assembled is key to understanding the intricacies of prokaryotic gene regulation. This article delves deep into the formation of the RNA polymerase holoenzyme, exploring its components, assembly mechanisms, and the significance of its structure in transcription initiation.
The Core Enzyme: The Foundation of RNA Polymerase
Before we discuss the holoenzyme, it's crucial to understand its foundation: the core enzyme. In Escherichia coli, the model prokaryote frequently studied in molecular biology, the core enzyme consists of five subunits:
-
Two α (alpha) subunits: These subunits are essential for enzyme assembly and interaction with regulatory proteins. They play a crucial role in the formation of the holoenzyme and contribute to the overall stability of the enzyme.
-
One β (beta) subunit: This subunit is responsible for binding ribonucleoside triphosphates (NTPs), the building blocks of RNA, and catalyzes the formation of phosphodiester bonds that link the nucleotides together during RNA synthesis. It's the catalytic powerhouse of the enzyme.
-
One β' (beta-prime) subunit: This subunit, along with the β subunit, forms the catalytic core and is responsible for binding the DNA template. Its interactions with the DNA are crucial for maintaining the stability of the transcription complex.
-
One ω (omega) subunit: This subunit plays a supportive role in enzyme assembly and stability, helping to correctly fold and maintain the structural integrity of the core enzyme. It isn't directly involved in catalysis or DNA binding.
The core enzyme, while capable of some RNA synthesis, lacks the specificity required for accurate transcription initiation at the correct promoters. This is where the sigma factor comes in.
The Sigma Factor: Specificity and Promoter Recognition
The sigma factor (σ) is a crucial subunit that converts the core enzyme into the RNA polymerase holoenzyme. This protein subunit doesn't participate directly in the catalytic process of RNA synthesis but provides the critical function of promoter recognition. Prokaryotes often have multiple sigma factors, each recognizing different promoter sequences, allowing for regulation of gene expression based on environmental conditions or developmental stages.
-
Promoter Recognition: The sigma factor interacts with specific DNA sequences upstream of the transcription start site, known as promoter sequences. These sequences typically contain a -10 region (Pribnow box) and a -35 region, highly conserved sequences that are recognized by the sigma factor. The interaction between the sigma factor and the promoter sequence is crucial for initiating transcription at the correct location on the DNA.
-
Types of Sigma Factors: E. coli, for example, possesses several sigma factors, each directing transcription of a different set of genes. The most commonly studied is σ<sup>70</sup>, which recognizes promoters for "housekeeping" genes – those required for normal cellular function under standard growth conditions. Other sigma factors, like σ<sup>32</sup> (heat shock response) or σ<sup>54</sup> (nitrogen metabolism), are expressed under specific stress conditions or during specific developmental stages, allowing the cell to adapt to changing environments.
-
Holoenzyme Formation: The binding of the sigma factor to the core enzyme is a highly regulated process. The α subunits of the core enzyme play a critical role in interacting with the sigma factor, initiating the formation of the holoenzyme complex. The precise details of this interaction are still being explored, but it is known to involve a series of conformational changes that bring the sigma factor into close proximity with the DNA-binding sites of the core enzyme.
The Holoenzyme: A Symphony of Subunits
The complete RNA polymerase holoenzyme is formed by the association of the core enzyme with a sigma factor. This complex is now fully equipped to initiate transcription at specific promoter sites. The holoenzyme possesses several significant advantages over the core enzyme:
-
Promoter Specificity: The most significant advantage of the holoenzyme is its ability to specifically recognize and bind to promoter regions. This ensures that transcription initiates at the correct location on the DNA, preventing the erroneous transcription of non-coding regions.
-
Accurate Initiation: The sigma factor not only facilitates promoter binding but also plays a vital role in the precise initiation of transcription. It helps to unwind the DNA double helix at the promoter region, creating the transcription bubble where RNA synthesis can begin.
-
Regulation of Gene Expression: The use of different sigma factors enables prokaryotes to finely regulate the expression of specific genes in response to environmental changes or developmental cues. This is a crucial mechanism for survival and adaptation.
-
Enhanced Stability: The sigma factor contributes to the overall stability of the RNA polymerase complex, ensuring that the enzyme remains functional during the lengthy process of transcription.
The Mechanism of Holoenzyme Formation: A Step-by-Step Look
While the precise details of holoenzyme assembly remain an active area of research, we have a good understanding of the key steps involved:
-
Core Enzyme Formation: Initially, the five subunits (α2ββ'ω) of the core enzyme self-assemble. This process involves a series of conformational changes and protein-protein interactions, which are guided by the specific amino acid sequences within the individual subunits. The ω subunit plays a crucial role in this process, assisting in the proper folding and assembly of the other subunits.
-
Sigma Factor Binding: Once the core enzyme is formed, the sigma factor (σ) binds to it. This binding occurs primarily through interactions between the sigma factor and the α subunits of the core enzyme. Specific amino acid residues on both proteins form non-covalent bonds, creating a stable complex. The sigma factor undergoes a conformational change upon binding, enabling it to engage with the promoter DNA.
-
Holoenzyme Completion: The successful binding of the sigma factor to the core enzyme completes the formation of the RNA polymerase holoenzyme (α2ββ'ωσ). This holoenzyme is now competent to bind to the promoter region of a gene and initiate transcription.
-
Promoter Binding: The holoenzyme, guided by the sigma factor, locates and binds to the promoter region of a gene. The sigma factor interacts with both the -10 and -35 regions, causing a localized unwinding of the DNA double helix, forming the open complex or transcription bubble.
-
Initiation of Transcription: Once the open complex is formed, the enzyme initiates RNA synthesis. The β subunit catalyzes the formation of phosphodiester bonds between ribonucleotides, using the DNA template strand as a guide.
-
Sigma Factor Release: After a few nucleotides have been added, the sigma factor typically dissociates from the core enzyme. The core enzyme then continues with elongation of the RNA molecule, moving along the DNA template, until it reaches a termination signal.
The Importance of Holoenzyme Formation in Gene Regulation
The formation of the RNA polymerase holoenzyme is not simply a structural event; it is a pivotal step in regulating gene expression. The specific sigma factor utilized by the cell determines which genes will be transcribed. This provides a sophisticated mechanism for adapting to changing conditions.
For example, during periods of heat stress, E. coli produces increased levels of σ<sup>32</sup>. This sigma factor directs the transcription of heat shock proteins, which help protect the cell from damage caused by elevated temperatures. Similarly, under nitrogen-limiting conditions, the expression of σ<sup>54</sup>-dependent genes involved in nitrogen metabolism is upregulated, allowing the cell to acquire necessary nitrogen sources.
The intricate interplay between core enzyme assembly, sigma factor binding, and promoter recognition underscores the remarkable elegance of prokaryotic gene regulation. The understanding of these processes is not only crucial for fundamental biological research but also has implications in developing strategies to combat bacterial infections and manipulating gene expression in biotechnological applications.
Future Directions and Research
Despite our current understanding, several aspects of RNA polymerase holoenzyme formation and function remain enigmatic. Ongoing research focuses on:
-
High-resolution structural studies: Determining the precise three-dimensional structures of the holoenzyme in complex with different sigma factors and promoter DNA will provide critical insights into the molecular mechanisms of promoter recognition and transcription initiation.
-
Understanding the dynamics of sigma factor binding and release: Investigating the conformational changes that occur during the binding and release of sigma factors will shed light on the regulatory mechanisms involved.
-
Exploring the roles of accessory factors: Several accessory proteins and chaperones are known to influence holoenzyme formation and activity. Further studies are needed to delineate their specific roles and interactions.
-
Comparative studies across diverse prokaryotes: Analyzing the diversity of RNA polymerases and sigma factors in different bacterial and archaeal species will broaden our understanding of the evolutionary adaptation of this essential transcription machinery.
By continuing to investigate these areas, scientists will further unravel the complexities of prokaryotic transcription and gain a deeper understanding of the remarkable precision and adaptability of this fundamental biological process. The ongoing research is crucial for advancing our knowledge of bacterial physiology, developing novel antibiotics, and pushing the boundaries of synthetic biology.
Latest Posts
Latest Posts
-
How Does Meiosis 1 Differ From Meiosis 2
Apr 26, 2025
-
The Heart Is Blank To The Spine
Apr 26, 2025
-
In Eukaryotic Cells Dna Is Found In
Apr 26, 2025
-
Which Molecule Has A Nonpolar Covalent Bond
Apr 26, 2025
-
Sources Of Water In The Desert
Apr 26, 2025
Related Post
Thank you for visiting our website which covers about In Prokaryotic Transcription How Is The Holoenzyme Rna Polymerase Formed . We hope the information provided has been useful to you. Feel free to contact us if you have any questions or need further assistance. See you next time and don't miss to bookmark.