In Rna Adenine Is Always Paired With
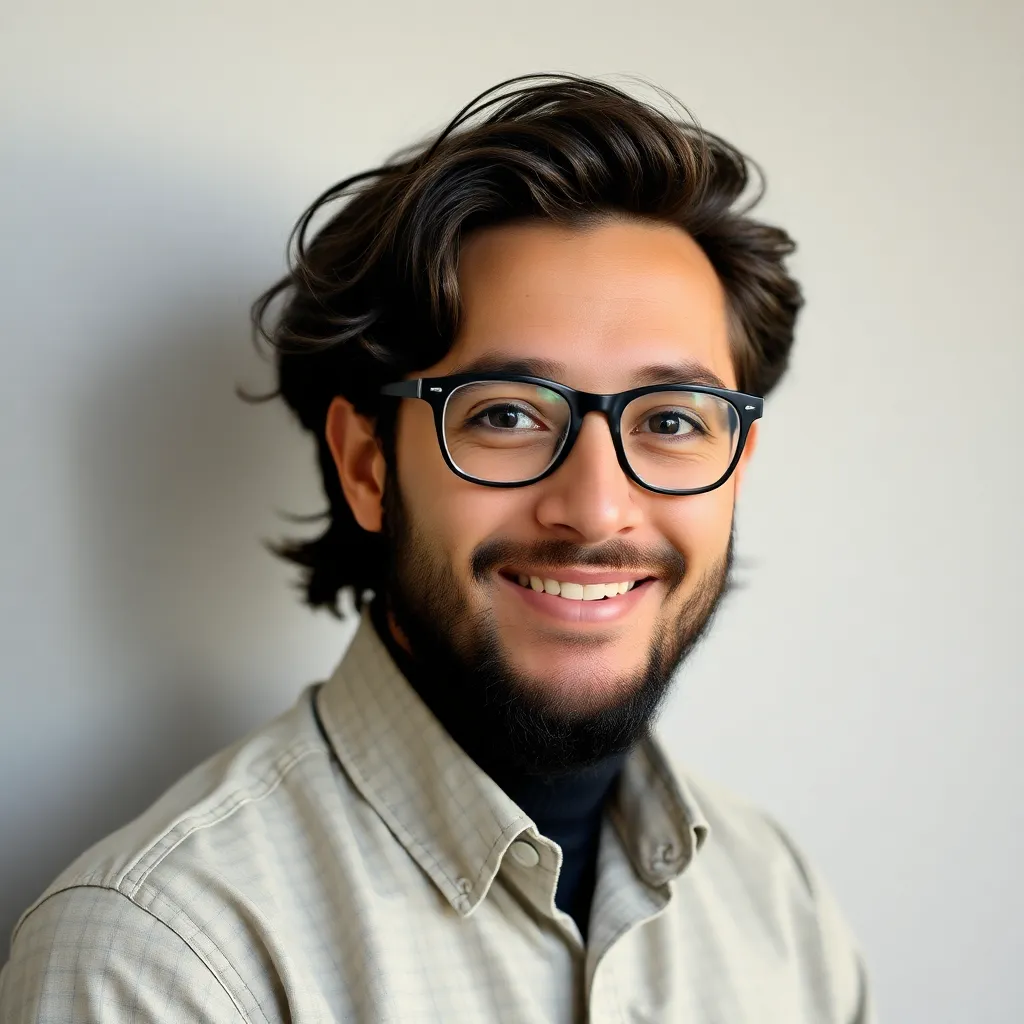
Muz Play
May 11, 2025 · 6 min read
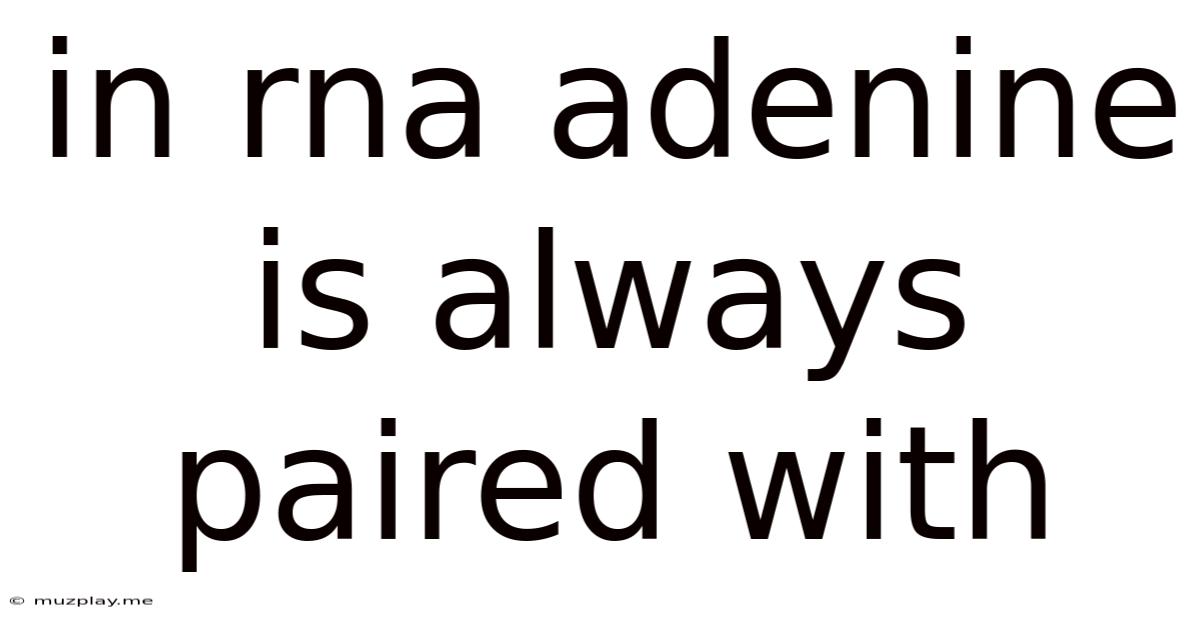
Table of Contents
In RNA, Adenine is Always Paired with… Uracil: Understanding RNA Structure and Function
RNA, or ribonucleic acid, is a crucial molecule in all forms of life, playing a vital role in protein synthesis and gene regulation. Unlike DNA, which uses thymine (T) to pair with adenine (A), RNA utilizes uracil (U) instead. Understanding this fundamental difference, along with the intricacies of RNA base pairing, is essential for comprehending the complex mechanisms of cellular processes. This comprehensive guide delves into the specifics of adenine-uracil pairing in RNA, exploring its implications for RNA structure, function, and various biological applications.
The Central Dogma and RNA's Role
The central dogma of molecular biology describes the flow of genetic information: DNA to RNA to protein. DNA, the primary repository of genetic information, serves as a template for RNA synthesis during transcription. This RNA molecule then carries the genetic code to the ribosome, where it directs protein synthesis through translation. This process relies heavily on the precise base pairing within RNA.
The Four RNA Nucleotides
RNA, like DNA, is composed of nucleotides. Each nucleotide consists of three components:
- A ribose sugar: A five-carbon sugar that forms the backbone of the RNA molecule. This is a key difference from DNA, which utilizes deoxyribose.
- A phosphate group: Connects the ribose sugars to create the phosphodiester backbone.
- A nitrogenous base: One of four possible bases: adenine (A), guanine (G), cytosine (C), and uracil (U).
RNA Base Pairing: The A-U Bond
In RNA, the base pairing follows a specific rule: adenine (A) always pairs with uracil (U), while guanine (G) always pairs with cytosine (C). This is achieved through hydrogen bonding. Adenine and uracil form two hydrogen bonds, a weaker bond compared to the three hydrogen bonds between guanine and cytosine. This difference in bond strength has implications for RNA secondary structure, as discussed below.
The Significance of A-U Pairing in RNA Structure
The A-U base pairing is critical in shaping the secondary and tertiary structures of RNA molecules. Unlike DNA, which typically exists as a double helix, RNA molecules often adopt complex three-dimensional structures that are crucial for their diverse functions.
Secondary Structure: Hairpins, Stem-Loops, and Bulges
The complementary base pairing between A and U (and G and C) allows RNA molecules to fold back on themselves, forming various secondary structures. These structures include:
- Hairpins: A single RNA strand folds back on itself, forming a stem (a double-stranded region due to base pairing) and a loop (a single-stranded region).
- Stem-loops: Similar to hairpins, but with a longer loop region.
- Bulges and internal loops: Regions within the double-stranded stem where one strand has extra unpaired bases.
These secondary structures are not merely random arrangements; they play crucial roles in RNA function. The specific arrangement of A-U and G-C base pairs determines the overall shape and stability of the RNA molecule. For instance, regions rich in A-U pairs are generally less stable due to the weaker hydrogen bonding, making these regions more flexible and prone to conformational changes.
Tertiary Structure: Complex 3D Arrangements
Further folding and interactions between different parts of the RNA molecule lead to the formation of complex tertiary structures. These structures often involve interactions between distant regions of the RNA, stabilized by hydrogen bonds, hydrophobic interactions, and stacking forces between the bases. The presence of A-U base pairs influences the overall tertiary structure by affecting the local flexibility and stability of the RNA molecule.
Functional Implications of A-U Base Pairing
The specific patterns of A-U and G-C base pairs are not randomly distributed within an RNA molecule. The arrangement is carefully orchestrated and crucial for the molecule’s function.
mRNA: Messenger RNA
Messenger RNA (mRNA) carries the genetic code from DNA to the ribosome. The precise sequence of A, U, G, and C nucleotides in mRNA determines the amino acid sequence of the resulting protein. While the overall structure of mRNA is largely linear, the presence of specific secondary structures can influence its translation efficiency and stability.
tRNA: Transfer RNA
Transfer RNA (tRNA) molecules play a crucial role in protein synthesis by bringing the correct amino acids to the ribosome during translation. tRNAs possess a characteristic cloverleaf secondary structure, stabilized by A-U and G-C base pairing. The specific arrangement of these base pairs is crucial for tRNA function, including its ability to recognize and bind to mRNA codons and aminoacyl-tRNA synthetases.
rRNA: Ribosomal RNA
Ribosomal RNA (rRNA) forms the structural and catalytic core of ribosomes. The precise folding of rRNA, determined by the specific base pairing patterns, is essential for the ribosome's function in protein synthesis. A-U base pairs contribute to the overall tertiary structure of rRNA, which includes complex interactions between different regions of the molecule.
Other Non-coding RNAs
Many other non-coding RNAs (ncRNAs) play diverse regulatory roles in gene expression. These ncRNAs, including microRNAs (miRNAs), small interfering RNAs (siRNAs), and long non-coding RNAs (lncRNAs), adopt specific secondary and tertiary structures essential for their functions. The arrangement of A-U and G-C pairs influences these structures and, consequently, their ability to interact with target molecules and regulate gene expression.
Evolutionary Significance of Uracil in RNA
The use of uracil in RNA instead of thymine in DNA is a significant evolutionary difference. While the reasons are not fully understood, some hypotheses suggest:
- Metabolic Cost: Uracil synthesis is energetically less expensive than thymine synthesis. This might have conferred an evolutionary advantage in early life forms.
- Deamination: Cytosine can spontaneously deaminate to uracil, a potentially harmful mutation. The presence of thymine in DNA allows for easier detection and repair of this mutation, as uracil is not a natural base in DNA. The lack of this repair mechanism in RNA might reflect its transient nature.
A-U Base Pairing in Research and Applications
Understanding the specifics of A-U base pairing has important implications for various research and applications.
RNA Structure Prediction
Computational methods are used to predict RNA secondary and tertiary structures based on the sequence of nucleotides. These methods consider the energetic contributions of A-U and G-C base pairs and other factors, such as stacking interactions, to accurately predict the 3D structure of RNA.
RNA Design and Engineering
Scientists can design and engineer RNA molecules with specific structures and functions by controlling the arrangement of A-U and G-C base pairs. This is crucial for developing therapeutic RNAs, such as antisense oligonucleotides and RNA interference (RNAi) molecules.
RNA-Based Therapeutics
RNA molecules are increasingly being used as therapeutics for various diseases. The precise design of these RNA molecules, including the strategic placement of A-U and G-C base pairs, is critical for their effectiveness and safety. For example, siRNAs and miRNAs are being developed as drugs targeting specific genes involved in disease pathogenesis.
Studying Gene Expression Regulation
Analyzing the A-U base pairing patterns in RNA molecules can reveal important information about gene expression regulation. Changes in the secondary structure of RNA, often resulting from alterations in A-U base pairing, can affect the stability, translation efficiency, or localization of RNA molecules.
Conclusion
The seemingly simple base pairing between adenine and uracil in RNA is a cornerstone of molecular biology. This fundamental interaction dictates RNA structure, influences its function, and plays a crucial role in diverse cellular processes. From protein synthesis to gene regulation, the intricacies of A-U pairing continue to fascinate and inform researchers, leading to groundbreaking advancements in various fields, including RNA-based therapeutics and gene editing. A thorough understanding of this interaction remains essential for unraveling the complexities of life itself.
Latest Posts
Latest Posts
-
What Is The Thermal Expansion Coefficient Of Steel
May 12, 2025
-
The Temple Of The Sun Machu Picchu
May 12, 2025
-
Domain And Range For Inverse Trig Functions
May 12, 2025
-
Chloroplasts Possess Circular Dna And Reproduce By Binary Fission
May 12, 2025
-
All Elements In This Group Have 1 Valence Electron
May 12, 2025
Related Post
Thank you for visiting our website which covers about In Rna Adenine Is Always Paired With . We hope the information provided has been useful to you. Feel free to contact us if you have any questions or need further assistance. See you next time and don't miss to bookmark.