Induced Fit Vs Lock And Key
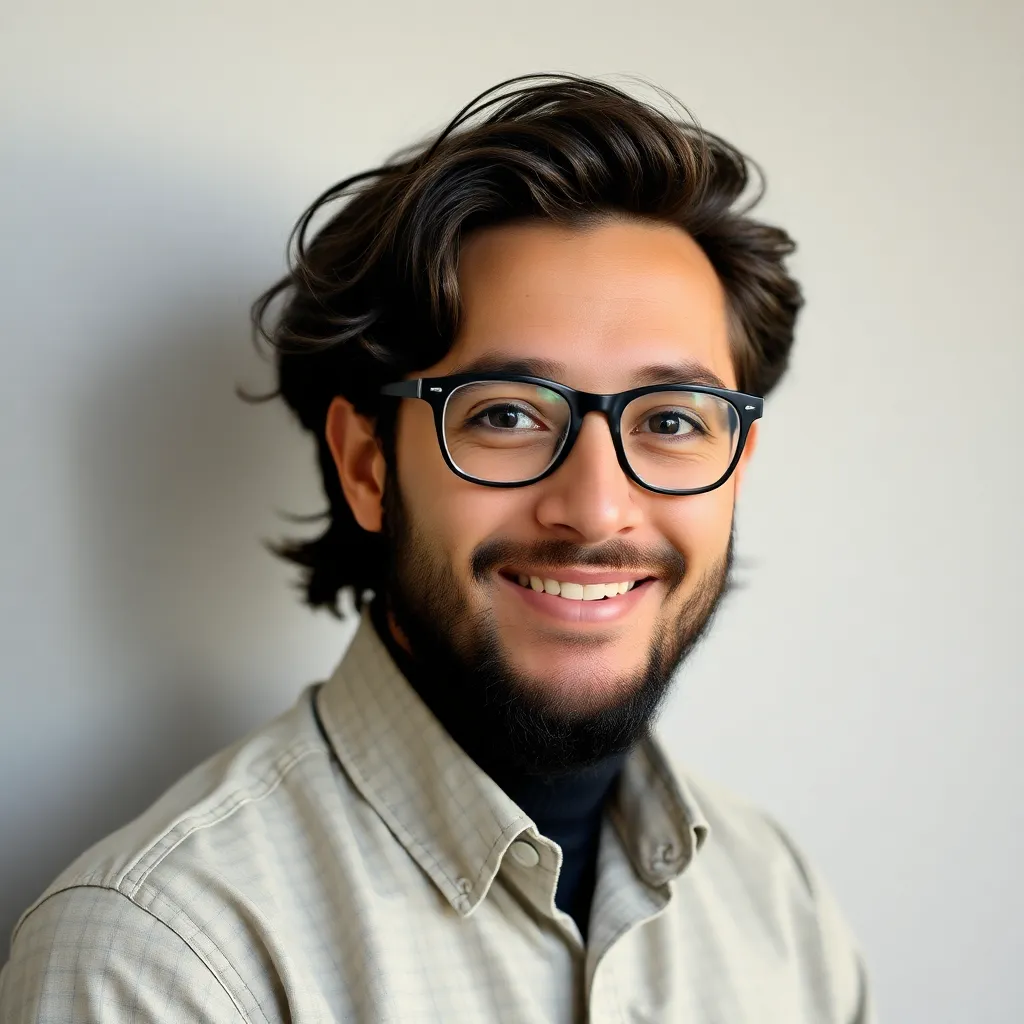
Muz Play
Apr 03, 2025 · 6 min read
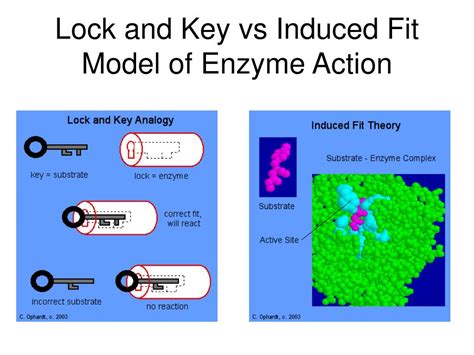
Table of Contents
Induced Fit vs. Lock and Key: A Deep Dive into Enzyme-Substrate Interactions
Enzyme-substrate interactions are fundamental to life itself. These interactions drive countless biochemical reactions, underpinning everything from digestion to DNA replication. Two dominant models have historically explained how enzymes bind to their substrates: the lock-and-key model and the induced-fit model. While the lock-and-key model provides a simplified initial understanding, the induced-fit model offers a more accurate and nuanced depiction of the dynamic process involved. This article will delve deep into both models, exploring their strengths, limitations, and the compelling evidence supporting the induced-fit model's current dominance.
The Lock-and-Key Model: A Simple Analogy
The lock-and-key model, proposed by Emil Fischer in 1894, presents a straightforward analogy. It likens the enzyme to a lock and the substrate to a key. The model posits that the enzyme possesses a rigid active site, a precisely shaped region complementary to the substrate's shape. Only the correctly shaped substrate can "fit" into the active site, much like a specific key fits into a specific lock. This binding initiates the catalytic process, leading to product formation.
Limitations of the Lock-and-Key Model
While elegantly simple, the lock-and-key model has significant limitations. It fails to account for several crucial observations:
- Enzyme flexibility: Enzymes are not rigid, static structures. They possess significant conformational flexibility, undergoing subtle changes in shape in response to various factors, including substrate binding.
- Transition state stabilization: The lock-and-key model doesn't adequately explain how enzymes stabilize the transition state, a high-energy intermediate formed during the reaction. Enzyme catalysis relies heavily on stabilizing this transition state, lowering the activation energy required for the reaction to proceed.
- Substrate diversity: Many enzymes can bind and process multiple substrates, often with varying degrees of efficiency. This broad substrate specificity is difficult to reconcile with a rigid, precisely shaped active site.
- Induced conformational changes: Experimental evidence overwhelmingly demonstrates that enzymes undergo significant conformational changes upon substrate binding. These changes are crucial for optimal substrate binding and catalysis.
The Induced-Fit Model: A More Realistic Picture
The induced-fit model, proposed by Daniel Koshland in 1958, provides a more accurate and dynamic representation of enzyme-substrate interactions. Unlike the rigid lock-and-key model, the induced-fit model suggests that the enzyme's active site is flexible and dynamic. The binding of the substrate induces a conformational change in the enzyme, causing the active site to adjust its shape to optimally accommodate the substrate.
Key Features of the Induced-Fit Model
The induced-fit model incorporates several key features that address the limitations of the lock-and-key model:
- Flexibility of the active site: The active site is not a pre-formed, rigid structure but rather a flexible region that adapts to the incoming substrate. This flexibility allows the enzyme to bind a range of substrates, albeit with varying affinities.
- Transition state stabilization: The conformational changes induced by substrate binding optimally position catalytic residues within the active site, facilitating the stabilization of the high-energy transition state. This stabilization significantly lowers the activation energy, accelerating the reaction rate.
- Substrate specificity: While the active site adjusts to the substrate, it still exhibits specificity. The specific interactions between the substrate and the enzyme's amino acid residues ensure that only compatible substrates can bind effectively.
- Enhanced catalysis: The induced fit not only improves substrate binding but also optimizes the orientation of catalytic residues, ensuring efficient catalysis. The conformational changes can also enhance the enzyme's ability to exclude water molecules, which might otherwise interfere with the reaction.
Experimental Evidence Supporting Induced Fit
Numerous experimental techniques provide compelling evidence supporting the induced-fit model:
- X-ray crystallography: High-resolution X-ray crystal structures of enzymes with and without bound substrates have revealed significant conformational changes in the active site upon substrate binding. These changes are often subtle but crucial for optimal substrate binding and catalysis.
- Nuclear magnetic resonance (NMR) spectroscopy: NMR spectroscopy offers dynamic information about protein structure and flexibility. Studies using NMR have demonstrated the conformational flexibility of enzymes and the induced fit upon substrate binding.
- Kinetic studies: Kinetic studies, which measure the rates of enzyme-catalyzed reactions, are consistent with the induced-fit model. The observed changes in reaction rates upon substrate binding align with the predicted conformational changes.
- Molecular dynamics simulations: Computer simulations employing molecular dynamics offer a powerful tool to visualize and analyze enzyme-substrate interactions at an atomic level. These simulations provide insights into the dynamic nature of enzyme-substrate binding and the conformational changes involved.
Specific Examples of Induced Fit
Several well-studied enzymes provide compelling examples of induced fit:
- Hexokinase: This enzyme catalyzes the transfer of a phosphate group from ATP to glucose. Upon glucose binding, the enzyme undergoes a large conformational change, effectively enclosing the substrate within the active site. This "induced closure" enhances the catalytic efficiency.
- Lysozyme: This enzyme cleaves bacterial cell walls. The substrate binding induces a conformational change that brings the catalytic residues into close proximity, facilitating the hydrolytic reaction.
- Trypsin: This protease cleaves peptide bonds. Substrate binding induces a conformational change that reorients catalytic residues, optimizing the catalytic process.
Induced Fit and Allosteric Regulation
The concept of induced fit extends beyond simple enzyme-substrate interactions. It plays a crucial role in allosteric regulation, where the binding of a molecule at one site on an enzyme affects its activity at a different site. The binding of an allosteric effector can induce conformational changes that either enhance or inhibit the enzyme's activity. This allows for fine-tuned control of enzyme activity in response to cellular signals.
Induced Fit: The Dominant Model
The overwhelming experimental evidence strongly supports the induced-fit model as the most accurate and comprehensive explanation for enzyme-substrate interactions. While the lock-and-key model provides a simplified introductory concept, it fails to capture the dynamic and adaptable nature of enzymes. The induced-fit model, with its emphasis on flexibility, conformational changes, and transition state stabilization, offers a far more complete and realistic picture of the intricate process by which enzymes catalyze biochemical reactions.
Implications for Drug Design
Understanding the induced-fit mechanism is crucial for drug discovery and design. Many drugs are designed to inhibit enzyme activity, and understanding the conformational changes associated with substrate binding and inhibition is critical for developing effective inhibitors. Rational drug design leverages this knowledge to create molecules that specifically target and bind to the enzyme's active site, disrupting its function and consequently inhibiting the enzyme's activity.
Conclusion: A Dynamic Partnership
The enzyme-substrate interaction is a dynamic partnership, far more complex than the static lock-and-key analogy initially suggested. The induced-fit model's emphasis on enzyme flexibility, conformational changes, and transition-state stabilization offers a significantly more accurate portrayal of this fundamental biological process. This understanding not only advances our fundamental knowledge of biochemistry but also holds significant implications for various fields, including drug discovery, biotechnology, and medical research. The continuous refinement of our understanding of induced fit, coupled with advancements in experimental techniques and computational modeling, promises to further enrich our knowledge of this crucial biological interaction. Further research will undoubtedly continue to unravel the intricate details of enzyme-substrate interactions, potentially leading to revolutionary advances in various scientific disciplines.
Latest Posts
Latest Posts
-
How Many Does Each Have Of 3
Apr 04, 2025
-
Why Does A Buffer Solution Resist Ph Changes
Apr 04, 2025
-
How To Multiply With Whole Numbers
Apr 04, 2025
-
What Is The Relationship Between Mass And Force
Apr 04, 2025
-
What Is The Difference In Meiosis 1 And 2
Apr 04, 2025
Related Post
Thank you for visiting our website which covers about Induced Fit Vs Lock And Key . We hope the information provided has been useful to you. Feel free to contact us if you have any questions or need further assistance. See you next time and don't miss to bookmark.