Introns Are Removed And Exons Are Spliced Together
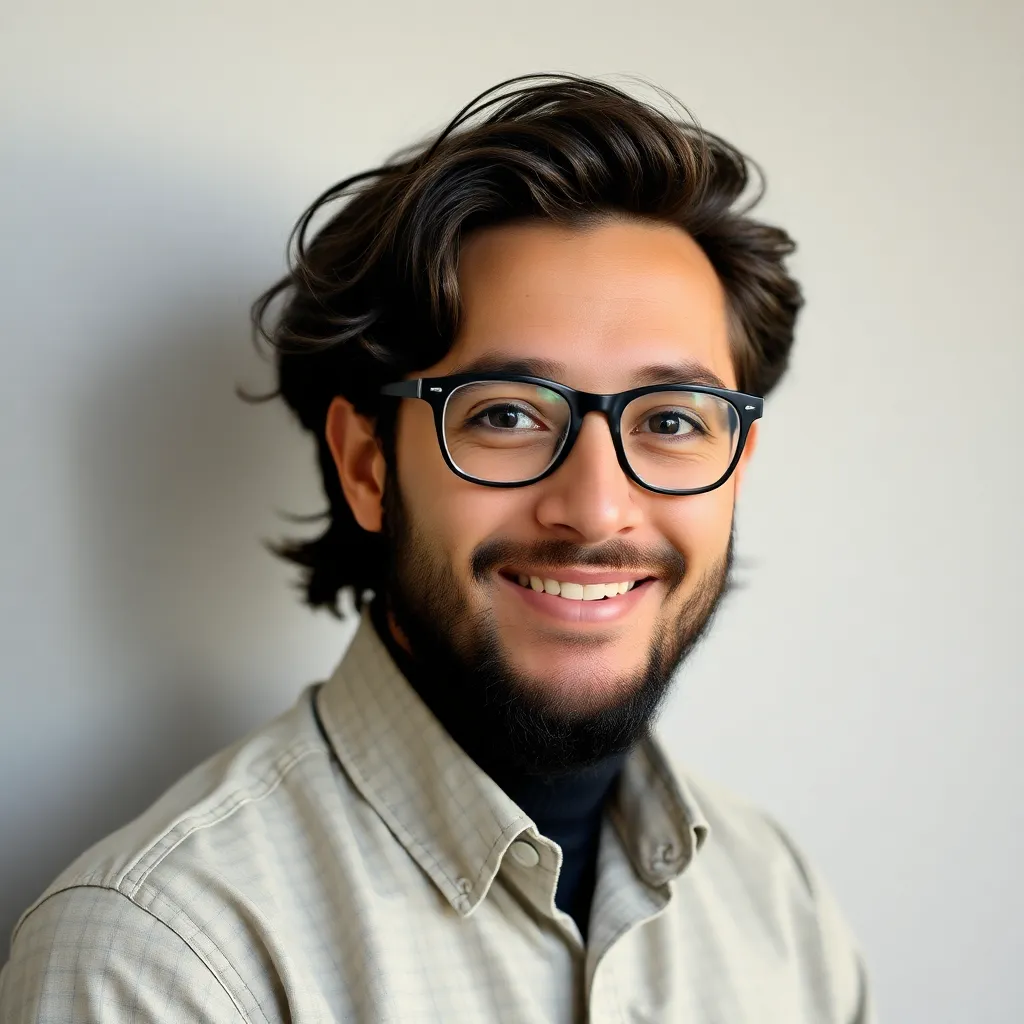
Muz Play
Mar 12, 2025 · 7 min read

Table of Contents
Introns are Removed and Exons are Spliced Together: Understanding RNA Splicing
The central dogma of molecular biology dictates that DNA is transcribed into RNA, which is then translated into protein. However, this process is far more nuanced than a simple linear progression. Eukaryotic genes contain regions called introns and exons. Introns are intervening sequences that are transcribed into RNA but are subsequently removed, while exons are expressed sequences that are joined together to form the mature messenger RNA (mRNA) molecule. This process of intron removal and exon joining, known as RNA splicing, is a crucial step in gene expression and is vital for the generation of functional proteins. A deeper understanding of this process is critical to comprehending various biological processes and human diseases.
What are Introns and Exons?
Before diving into the mechanics of splicing, let's define the key players:
Introns: The Intervening Sequences
Introns are non-coding sequences within a gene that interrupt the coding sequences (exons). They are transcribed into pre-mRNA but are not translated into protein. These sequences were initially considered "junk DNA," but research has revealed that introns play significant roles in gene regulation, alternative splicing, and genome evolution. Their presence allows for greater complexity in gene expression and protein diversity. The lengths of introns vary widely, ranging from a few dozen nucleotides to tens of thousands.
Exons: The Expressed Sequences
Exons, in contrast to introns, are the coding sequences of a gene. They are transcribed into pre-mRNA and ultimately translated into the amino acid sequence of a protein. Exons are responsible for determining the protein's structure and function. While exons often encode for specific protein domains or functional units, their arrangement and combination significantly influence the final protein product.
The Mechanism of RNA Splicing: A Precise Molecular Ballet
RNA splicing is a remarkably precise process, removing introns with remarkable accuracy and joining exons seamlessly. The main machinery responsible for this feat is the spliceosome, a large and dynamic ribonucleoprotein complex composed of five small nuclear ribonucleoproteins (snRNPs: U1, U2, U4, U5, and U6) and numerous protein factors.
The Spliceosome: The Master Conductor
The spliceosome’s function is akin to a highly specialized molecular machine. It recognizes specific sequences within the pre-mRNA, cuts the RNA at the intron-exon boundaries, removes the introns, and ligates (joins) the exons together. This process involves several key steps:
1. Splice Site Recognition: The spliceosome identifies the intron-exon boundaries, which are marked by specific consensus sequences. These sequences, including the 5' splice site (GU), the branch point (A), and the 3' splice site (AG), are critical for accurate splicing.
2. Intron Loop Formation: After recognition, the spliceosome catalyzes the formation of a lariat structure, a loop formed by joining the 5' end of the intron to the branch point adenine (A) residue within the intron. This loop formation effectively isolates the intron from the exons.
3. Exon Junction: Once the lariat is formed, the spliceosome cleaves the RNA at the 3' splice site, releasing the intron lariat and joining the two adjacent exons together. The joined exons create a continuous coding sequence.
4. Lariat Degradation: The intron lariat is then degraded, ensuring that it doesn't interfere with further protein synthesis or other cellular processes.
Alternative Splicing: Expanding the Proteome
One of the most remarkable aspects of RNA splicing is its ability to generate multiple protein isoforms from a single gene through a process called alternative splicing. This occurs when different combinations of exons are joined together, resulting in different mRNA molecules and ultimately, different protein products. Alternative splicing dramatically increases the proteome's complexity, allowing a single gene to encode for multiple proteins with diverse functions. This mechanism is crucial for cellular differentiation, tissue-specific gene expression, and adaptation to environmental changes.
For example, a gene with five exons (exon 1, 2, 3, 4, and 5) could undergo alternative splicing to produce mRNA molecules containing various exon combinations (e.g., 1-2-3-4-5, 1-2-4-5, 1-3-5). This leads to the synthesis of different proteins, each with its unique properties and functions.
The Importance of Accurate Splicing
The accuracy of RNA splicing is paramount for proper gene expression and cellular function. Errors in splicing, such as exon skipping or intron retention, can lead to the production of non-functional or truncated proteins. These errors have been implicated in various human diseases, including cancer, neurodegenerative disorders, and genetic disorders.
Consequences of Splicing Errors: A Cascade of Problems
Splicing errors can trigger several detrimental consequences:
- Non-functional proteins: Incorrectly spliced mRNA molecules often lead to the production of proteins with altered amino acid sequences, resulting in loss of function or gain of toxic functions.
- Truncated proteins: Premature stop codons resulting from splicing errors can lead to truncated proteins lacking essential functional domains.
- Disease development: Accumulation of non-functional or misfolded proteins can disrupt cellular processes, triggering cellular stress, apoptosis (programmed cell death), and ultimately, the development of various diseases.
Splicing Factors and Regulation
The process of RNA splicing isn't simply a passive event; it's tightly regulated by various splicing factors. These factors, including splicing enhancers and splicing silencers, can influence the choice of splice sites and contribute to alternative splicing patterns. These factors bind to specific sequences within the pre-mRNA, either promoting or inhibiting the inclusion of particular exons in the mature mRNA.
The Role of Splicing Factors in Disease
Mutations affecting splicing factors or the regulatory sequences involved in splicing can disrupt the normal splicing process, leading to disease. For example, mutations in spliceosomal proteins have been linked to various cancers and inherited diseases.
RNA Splicing and its Clinical Significance
Understanding RNA splicing is essential for diagnosing and treating human diseases. Mis-splicing is a significant contributor to many genetic disorders, making the study of splicing critical for developing therapeutic interventions.
Splicing and Cancer: A Tumorous Interplay
Aberrant splicing patterns are frequently observed in various types of cancer. These abnormal splicing events can lead to the production of oncogenic proteins (proteins that promote cancer development) or the loss of tumor suppressor proteins (proteins that inhibit cancer development).
Splicing and Genetic Disorders: A Complex Relationship
Many genetic diseases are caused by mutations affecting splicing signals or splice sites. These mutations can alter the splicing process, resulting in the production of non-functional or truncated proteins.
Studying RNA Splicing: Techniques and Approaches
Investigating the intricacies of RNA splicing requires specialized techniques:
- RT-PCR (Reverse Transcription Polymerase Chain Reaction): This technique allows researchers to detect and quantify different splice isoforms of a gene.
- Next-generation sequencing (NGS): NGS provides a high-throughput approach for identifying and characterizing splicing events on a genome-wide scale.
- Bioinformatics analysis: Computational approaches are essential for analyzing large datasets generated by NGS, predicting splice sites, and identifying splicing regulatory elements.
Future Directions: The Ever-Evolving Field of RNA Splicing
The field of RNA splicing continues to evolve rapidly. Ongoing research focuses on several key areas:
- Identifying novel splicing regulatory elements: Understanding the regulatory mechanisms governing splicing is essential for developing targeted therapies for splicing-related diseases.
- Developing splicing-modulating therapies: Researchers are actively developing novel therapeutic strategies to correct aberrant splicing patterns in disease.
- Investigating the role of RNA splicing in development and evolution: Understanding the evolutionary significance of alternative splicing can provide insights into the diversity and complexity of life.
In conclusion, the removal of introns and the splicing together of exons is a fundamental and highly regulated process critical for gene expression and protein synthesis. The accuracy of this process is crucial for maintaining cellular health and preventing the development of diseases. Further research into the intricacies of RNA splicing will undoubtedly unveil new mechanisms and potential therapeutic targets for a wide range of human diseases, solidifying its place as a critical area of study in biology and medicine. This intricate molecular ballet underscores the astonishing complexity and elegance of the cellular machinery that underpins all of life.
Latest Posts
Latest Posts
-
How Does Electronegativity Affect Interactions Between Water Molecules
May 09, 2025
-
Using Ksp To Calculate The Solubility Of A Compound
May 09, 2025
-
Is Ethyl Alcohol Acidic Or Basic
May 09, 2025
-
When Is Co2 Released In Cellular Respiration
May 09, 2025
-
Which Problem Can Be Solved By Division Of Decimals
May 09, 2025
Related Post
Thank you for visiting our website which covers about Introns Are Removed And Exons Are Spliced Together . We hope the information provided has been useful to you. Feel free to contact us if you have any questions or need further assistance. See you next time and don't miss to bookmark.