Part B - The Replication Fork
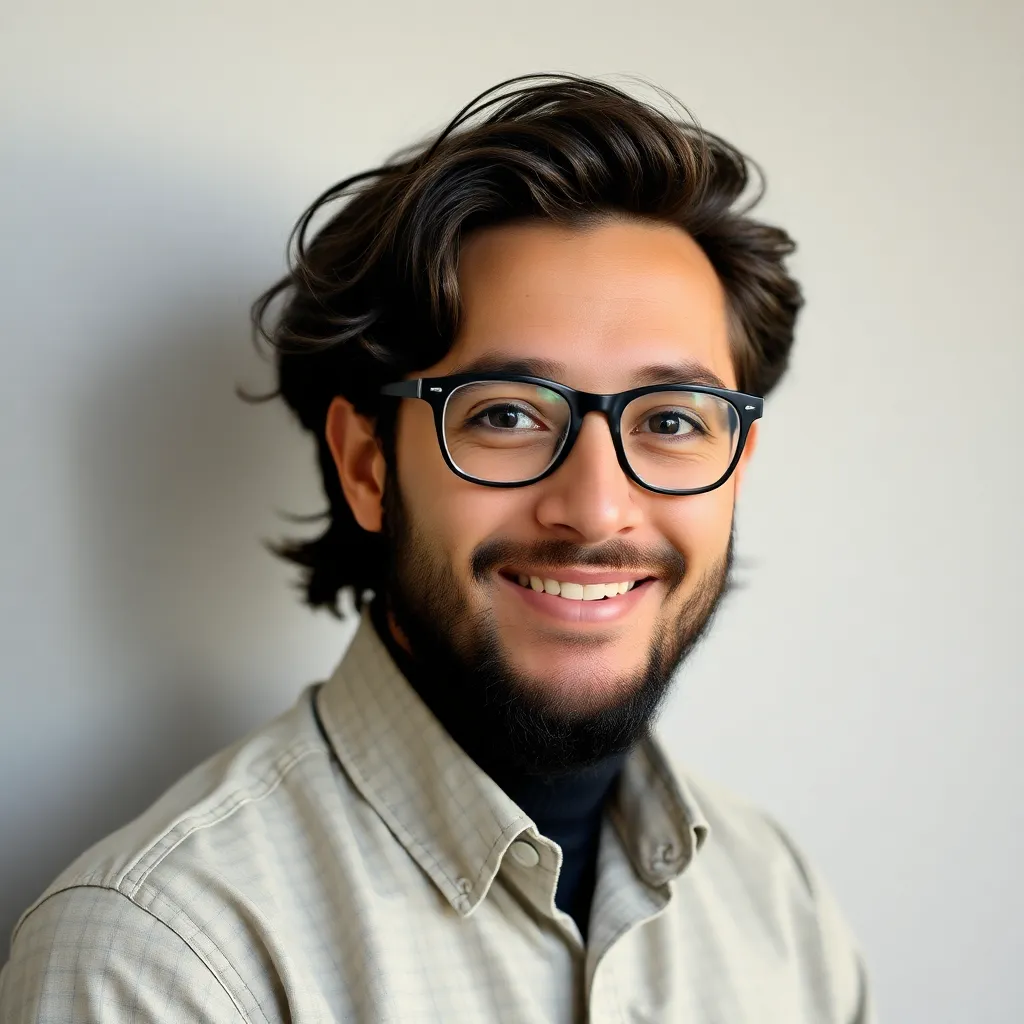
Muz Play
Apr 18, 2025 · 7 min read

Table of Contents
Part B: The Replication Fork – A Deep Dive into DNA Replication's Engine
DNA replication, the process by which a cell creates an exact copy of its genome, is a fundamental process for life. Understanding this intricate process is crucial for comprehending cellular growth, development, and the mechanisms behind genetic diseases. This article delves into the heart of DNA replication: the replication fork, the dynamic structure where the double helix unwinds and new strands are synthesized.
The Replication Fork: Structure and Function
The replication fork is a Y-shaped structure formed during DNA replication. It's the site where the parental DNA double helix unwinds, and new strands are synthesized using the parental strands as templates. This isn't a static structure; it's a highly dynamic complex involving numerous proteins working in concert. Let's break down its key components:
1. Parental DNA Double Helix: The Template
The replication process begins with the parental DNA double helix, which is the template for the synthesis of new DNA strands. The double helix needs to be unwound to expose the bases, which allows for the formation of complementary base pairs during replication. This unwinding is not a passive process; it requires the activity of specific enzymes.
2. Helicase: Unwinding the Helix
Helicase is a crucial enzyme that plays the role of "unzipper" in DNA replication. It's responsible for unwinding the DNA double helix at the replication fork by breaking the hydrogen bonds between the base pairs. This unwinding creates two single-stranded DNA templates, ready for the synthesis of new strands. The unwinding process is energetically demanding, requiring ATP hydrolysis.
3. Single-Stranded Binding Proteins (SSBs): Stabilizing the Templates
Once the helicase unwinds the DNA, the single-stranded DNA (ssDNA) is vulnerable to re-annealing (reforming the double helix) or forming secondary structures that could interfere with replication. Single-stranded binding proteins (SSBs) bind to the separated strands, preventing them from re-annealing and stabilizing them for the action of other replication proteins. SSBs bind cooperatively, meaning the binding of one SSB molecule facilitates the binding of others.
4. Topoisomerase: Relieving Torsional Strain
The unwinding of the DNA helix by helicase introduces torsional stress ahead of the replication fork. This stress, if left unchecked, can cause the DNA to supercoil and prevent further unwinding. Topoisomerases are enzymes that relieve this torsional stress by cutting and rejoining DNA strands. They prevent the build-up of supercoiling and ensure the smooth progression of the replication fork. Type I topoisomerases cut one strand, while Type II topoisomerases cut both strands.
5. Primase: Synthesizing RNA Primers
DNA polymerases, the enzymes responsible for synthesizing new DNA strands, cannot initiate DNA synthesis de novo. They require a pre-existing 3'-OH group to add nucleotides to. This is where primase comes into play. Primase is an RNA polymerase that synthesizes short RNA primers complementary to the template DNA strands. These RNA primers provide the 3'-OH group necessary for DNA polymerase to start DNA synthesis.
6. DNA Polymerase: The Master Builders
DNA polymerases are the workhorses of DNA replication. These enzymes catalyze the addition of deoxyribonucleotides to the 3'-OH end of the growing DNA strand, using the template strand as a guide. Different DNA polymerases have different roles in replication. For instance, in E. coli, DNA polymerase III is the main replicative polymerase, while DNA polymerase I is involved in primer removal and gap filling. Eukaryotes have multiple DNA polymerases with specialized functions.
- DNA Polymerase III (prokaryotes) / α, δ, ε (eukaryotes): Responsible for the bulk of DNA synthesis.
- DNA Polymerase I (prokaryotes) / β, γ (eukaryotes): Involved in repair and primer removal.
7. Leading and Lagging Strands: Different Modes of Synthesis
DNA polymerase can only synthesize DNA in the 5' to 3' direction. Since the parental DNA strands are antiparallel, DNA synthesis occurs differently on each strand:
-
Leading Strand: Synthesized continuously in the 5' to 3' direction, following the replication fork. Only one primer is needed for the entire leading strand synthesis.
-
Lagging Strand: Synthesized discontinuously in short fragments called Okazaki fragments. Each Okazaki fragment requires its own RNA primer. The lagging strand synthesis proceeds in the opposite direction of the replication fork movement.
8. DNA Ligase: Joining Okazaki Fragments
The discontinuous synthesis of the lagging strand results in a series of Okazaki fragments. DNA ligase is the enzyme that joins these fragments together, creating a continuous lagging strand. DNA ligase forms a phosphodiester bond between the 3'-OH end of one Okazaki fragment and the 5'-phosphate end of the next, creating a seamless DNA molecule.
9. Sliding Clamp: Enhancing Processivity
DNA polymerases need to be highly processive, meaning they need to synthesize long stretches of DNA without frequently detaching from the template. The sliding clamp is a ring-shaped protein that encircles the DNA and keeps the DNA polymerase firmly attached to the template strand, greatly increasing its processivity.
10. Clamp Loader: Assembling the Sliding Clamp
The sliding clamp needs to be loaded onto the DNA template, and this is the job of the clamp loader. This protein complex uses ATP hydrolysis to open the sliding clamp and load it onto the DNA at the primer-template junction.
Regulation and Fidelity of Replication
The replication fork isn't just a collection of enzymes working independently; it's a highly regulated and coordinated machine. Several mechanisms ensure the accuracy and efficiency of DNA replication:
Proofreading Activity of DNA Polymerase
DNA polymerases possess proofreading activity, a 3' to 5' exonuclease activity that removes incorrectly incorporated nucleotides. This ensures high fidelity during DNA replication. If an incorrect nucleotide is added, the polymerase can backtrack, remove the wrong nucleotide, and replace it with the correct one.
Mismatch Repair System
Despite the proofreading activity of DNA polymerases, some errors can escape detection. The mismatch repair (MMR) system detects and corrects these errors after replication. MMR proteins recognize mismatched base pairs and initiate a repair process that involves excising the incorrect nucleotide and replacing it with the correct one.
Telomere Replication: Protecting the Ends
Linear chromosomes present a unique challenge for replication. The lagging strand cannot be fully replicated at the ends, leading to the shortening of chromosomes with each replication cycle. Telomeres, repetitive DNA sequences at the ends of chromosomes, and the enzyme telomerase help prevent this shortening. Telomerase extends the telomeres, preventing the loss of essential genetic information.
Variations and Exceptions
While the basic principles of replication fork function are conserved across organisms, variations exist:
-
Prokaryotic vs. Eukaryotic Replication: Prokaryotes have a single origin of replication, while eukaryotes have multiple origins. This difference reflects the size and complexity of their genomes.
-
Rolling Circle Replication: A specialized type of replication used by some viruses and plasmids, where replication proceeds in a circular fashion without the formation of a traditional replication fork.
-
Replication of Damaged DNA: Specialized mechanisms exist to replicate DNA containing damaged bases or lesions, ensuring genomic stability even in the face of DNA damage.
Clinical Significance
The replication fork is a critical target for various anticancer drugs. Many chemotherapeutic agents target enzymes involved in DNA replication, such as topoisomerases and DNA polymerases, disrupting the process and leading to cell death. Understanding the intricacies of the replication fork is essential for developing novel cancer therapies. Furthermore, defects in the replication fork machinery can lead to genomic instability and contribute to genetic diseases.
Conclusion
The replication fork is a marvel of biological engineering, a highly dynamic and coordinated machine that ensures the faithful duplication of the genome. Its intricate workings, involving numerous proteins and complex regulatory mechanisms, highlight the precision and robustness of cellular processes. Further research into the replication fork is crucial not only for expanding our fundamental understanding of biology but also for developing new therapeutic strategies for treating diseases linked to DNA replication defects. From the unwinding of the double helix by helicase to the final ligation of Okazaki fragments by ligase, each component plays a critical role in the fidelity and efficiency of this essential process. The ongoing study of the replication fork continues to yield valuable insights into the mechanisms that maintain the integrity and stability of our genetic material.
Latest Posts
Latest Posts
-
The First Electron Acceptor Of Cellular Respiration Is
Apr 19, 2025
-
Glycolysis Cannot Occur In An Anaerobic Environment
Apr 19, 2025
-
Can A Quadratic Function Be Periodic
Apr 19, 2025
-
Is Tungsten A Metal Nonmetal Or Metalloid
Apr 19, 2025
-
Electric Field Of Uniformly Charged Sphere
Apr 19, 2025
Related Post
Thank you for visiting our website which covers about Part B - The Replication Fork . We hope the information provided has been useful to you. Feel free to contact us if you have any questions or need further assistance. See you next time and don't miss to bookmark.