Polymer Of Amino Acids Are Called
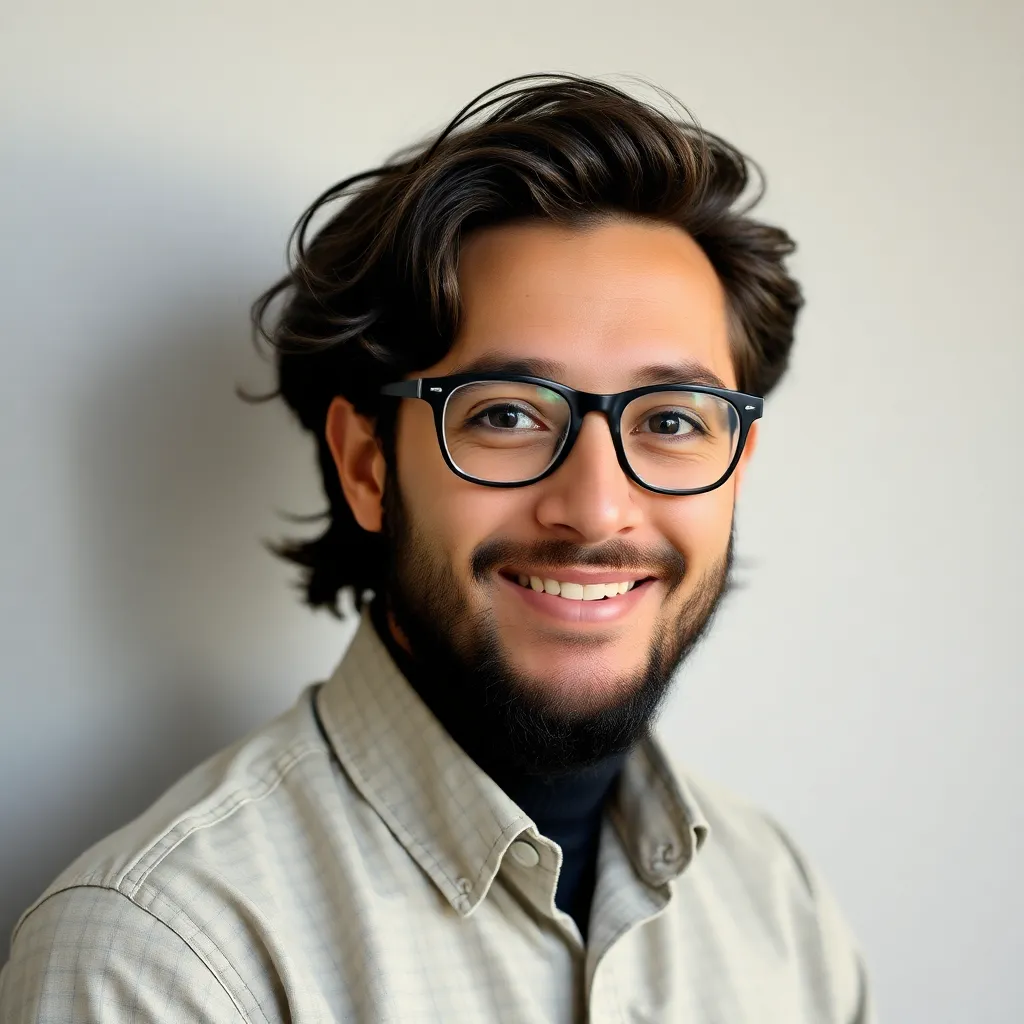
Muz Play
Apr 06, 2025 · 6 min read

Table of Contents
Polymers of Amino Acids are Called Proteins: A Deep Dive into the Building Blocks of Life
Polymers of amino acids are called proteins. These aren't just random chains; proteins are incredibly complex and vital biomolecules that perform a vast array of functions essential to life. From catalyzing biochemical reactions to providing structural support, proteins are the workhorses of the cell. Understanding their structure, function, and synthesis is crucial to grasping the intricacies of biology.
What are Amino Acids?
Before delving into the complexities of proteins, it's vital to understand their fundamental building blocks: amino acids. Amino acids are organic molecules containing both an amino group (-NH2) and a carboxyl group (-COOH). This seemingly simple structure is surprisingly versatile. The key to this versatility lies in the side chain (R group), which varies significantly among the 20 standard amino acids. This side chain dictates the amino acid's properties, influencing its polarity, charge, and hydrophobicity.
The 20 Standard Amino Acids: A Diverse Cast of Characters
The 20 standard amino acids, encoded by the genetic code, can be categorized based on their side chain properties:
-
Nonpolar, aliphatic amino acids: These possess hydrocarbon side chains, making them hydrophobic (water-repelling). Examples include glycine, alanine, valine, leucine, isoleucine, and methionine.
-
Aromatic amino acids: These have ring structures in their side chains, often contributing to their absorbance of ultraviolet light. Phenylalanine, tyrosine, and tryptophan are examples.
-
Polar, uncharged amino acids: These have polar but uncharged side chains, leading to interactions with water molecules. Serine, threonine, cysteine, asparagine, and glutamine fall into this category. Cysteine is unique due to its ability to form disulfide bonds, contributing significantly to protein structure.
-
Positively charged (basic) amino acids: These have positively charged side chains at physiological pH. Lysine, arginine, and histidine are prime examples.
-
Negatively charged (acidic) amino acids: These possess negatively charged side chains at physiological pH. Aspartate and glutamate are the representatives.
The diversity of these amino acids allows for a vast array of protein structures and functions. The specific sequence of amino acids in a protein, known as its primary structure, determines its final three-dimensional shape and, consequently, its function.
The Levels of Protein Structure: From Linear Chain to Functional Fold
The remarkable functionality of proteins arises from their intricate three-dimensional structures. These structures are hierarchical, encompassing four levels of organization:
1. Primary Structure: The Amino Acid Sequence
The primary structure is simply the linear sequence of amino acids linked together by peptide bonds. These peptide bonds are formed through a dehydration reaction between the carboxyl group of one amino acid and the amino group of another. The primary structure dictates all higher-order structures. Even a single amino acid change can drastically alter a protein's function, as seen in the case of sickle cell anemia, where a single amino acid substitution in hemoglobin leads to a dysfunctional protein.
2. Secondary Structure: Local Folding Patterns
The secondary structure refers to local folding patterns within the polypeptide chain. These patterns are stabilized by hydrogen bonds between the backbone amide and carbonyl groups. The most common secondary structures are:
-
Alpha-helices: A right-handed coil stabilized by hydrogen bonds between every fourth amino acid.
-
Beta-sheets: Extended polypeptide chains arranged side-by-side, stabilized by hydrogen bonds between adjacent strands. These can be parallel or antiparallel, depending on the orientation of the strands.
-
Turns and loops: These are short, irregular segments that connect alpha-helices and beta-sheets, contributing to the overall protein fold.
3. Tertiary Structure: The Three-Dimensional Arrangement
The tertiary structure describes the overall three-dimensional arrangement of a polypeptide chain, including the spatial relationships between its secondary structure elements. This structure is primarily stabilized by interactions between the amino acid side chains, including:
-
Hydrophobic interactions: Nonpolar side chains cluster together in the protein's interior, minimizing their contact with water.
-
Hydrogen bonds: Polar side chains form hydrogen bonds with each other or with water molecules.
-
Ionic bonds (salt bridges): Attractive forces between oppositely charged side chains.
-
Disulfide bonds: Covalent bonds between cysteine residues, creating strong cross-links within the protein.
The tertiary structure is crucial for a protein's function. The specific three-dimensional arrangement brings together amino acid residues to form active sites (in enzymes) or binding pockets (in receptors).
4. Quaternary Structure: The Assembly of Subunits
Some proteins consist of multiple polypeptide chains, or subunits, assembled together to form a functional protein complex. This arrangement is called the quaternary structure. The subunits can be identical or different, and their interactions are stabilized by the same forces that stabilize tertiary structure. Hemoglobin, for example, is a tetramer composed of four subunits.
Protein Synthesis: From Gene to Functional Protein
The synthesis of proteins is a complex, tightly regulated process involving two major steps:
1. Transcription: DNA to mRNA
The genetic information encoding a protein resides in DNA. Transcription is the process of copying this information into a messenger RNA (mRNA) molecule. This mRNA molecule then carries the genetic code from the nucleus to the ribosomes, the protein synthesis machinery of the cell.
2. Translation: mRNA to Protein
Translation is the process of decoding the mRNA sequence into a polypeptide chain. Ribosomes read the mRNA sequence in codons (three-nucleotide units), each codon specifying a particular amino acid. Transfer RNA (tRNA) molecules, each carrying a specific amino acid, bind to their corresponding codons on the mRNA, delivering the amino acids to the ribosome. The ribosome then catalyzes the formation of peptide bonds between the amino acids, building the polypeptide chain according to the mRNA sequence.
Protein Function: A Multitude of Roles
Proteins perform a vast array of functions essential to life, including:
-
Enzymes: Catalyze biochemical reactions, speeding up the rate of reactions without being consumed themselves.
-
Structural proteins: Provide structural support and shape to cells and tissues, such as collagen and keratin.
-
Transport proteins: Carry molecules across cell membranes or throughout the body, such as hemoglobin and membrane transporters.
-
Motor proteins: Generate movement, such as myosin in muscles and kinesin in intracellular transport.
-
Hormones: Chemical messengers that regulate various physiological processes.
-
Receptors: Bind to specific molecules and trigger cellular responses.
-
Antibodies: Part of the immune system, recognizing and neutralizing foreign substances.
Protein Misfolding and Disease
The correct folding of proteins is crucial for their function. Misfolded proteins can lead to a variety of diseases, including:
-
Alzheimer's disease: Associated with the aggregation of amyloid-beta peptides.
-
Parkinson's disease: Linked to the accumulation of alpha-synuclein aggregates.
-
Prion diseases: Caused by misfolded prion proteins that induce misfolding of other prion proteins.
-
Cystic fibrosis: Caused by a mutation in the CFTR protein, leading to misfolding and dysfunction.
Understanding protein folding and misfolding is crucial for developing therapies for these diseases.
Conclusion: The Remarkable World of Proteins
Polymers of amino acids, or proteins, are incredibly diverse and essential biomolecules. Their complex structures and functions underpin nearly every aspect of life. From the intricate folding patterns that determine their activity to the vast array of roles they play, proteins are a testament to the elegance and complexity of biological systems. Continued research into protein structure, function, and synthesis promises to reveal even more about the fundamental processes of life and to provide new insights into the treatment of diseases. The field continues to expand, with new techniques and technologies providing deeper understanding of this crucial class of biomolecules. Further exploration into post-translational modifications, protein-protein interactions, and proteomics will further illuminate the intricate world of proteins and their vital contributions to biological systems. The ongoing quest to understand these remarkable molecules holds the key to unlocking many mysteries of life itself.
Latest Posts
Latest Posts
-
Temperature Range Of The Inner Core
Apr 07, 2025
-
Does Plant And Animal Cells Have Cytoplasm
Apr 07, 2025
-
Electron Dot Diagram For Periodic Table
Apr 07, 2025
-
Respiratory Centers Are Located In The Pons
Apr 07, 2025
-
What Is Unusual About Glutathiones Structure
Apr 07, 2025
Related Post
Thank you for visiting our website which covers about Polymer Of Amino Acids Are Called . We hope the information provided has been useful to you. Feel free to contact us if you have any questions or need further assistance. See you next time and don't miss to bookmark.