Report Sheet Lab 7 Electron Dot Structures And Molecular Shape
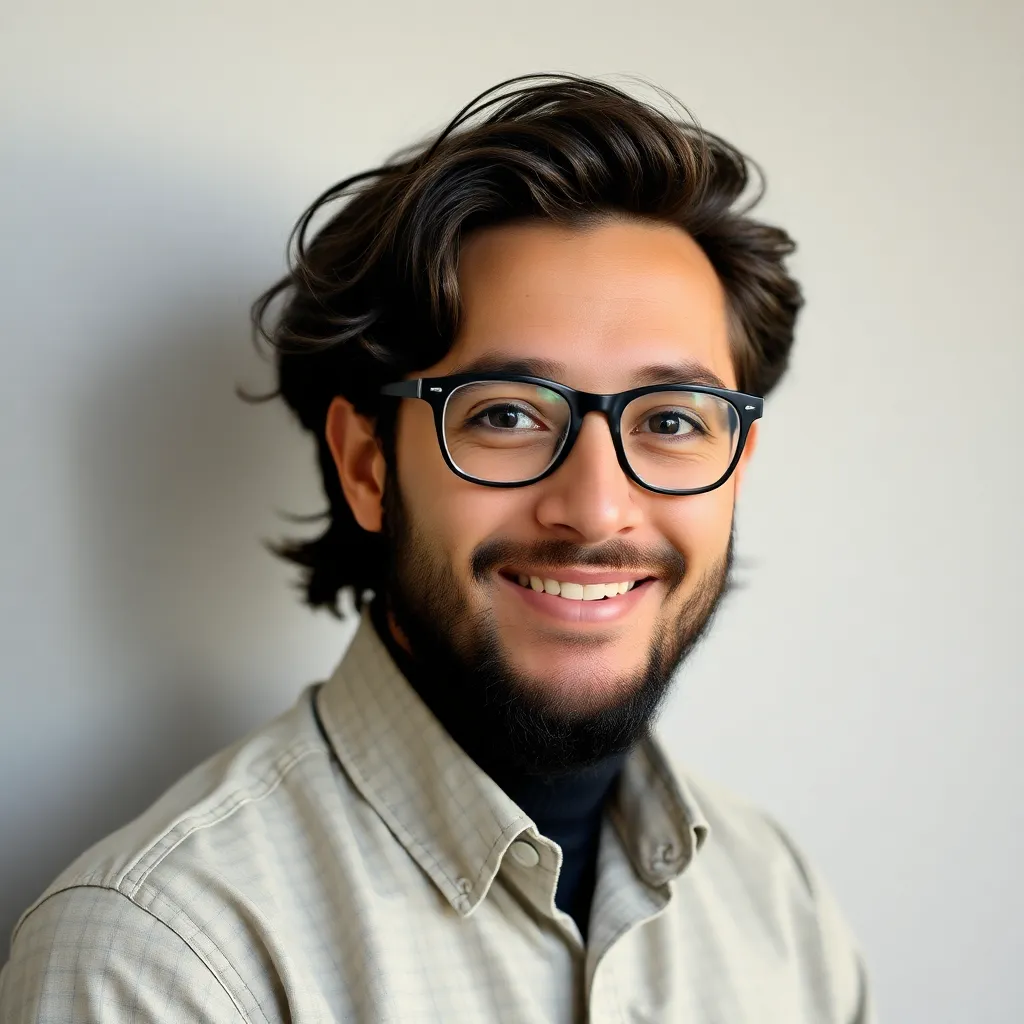
Muz Play
May 10, 2025 · 7 min read
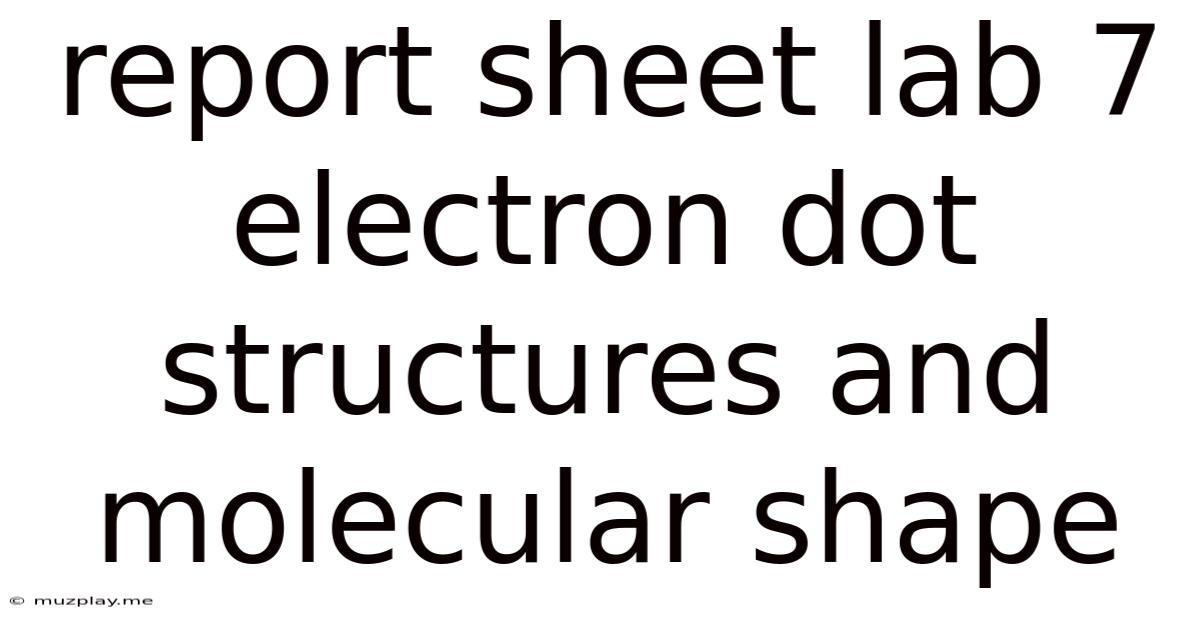
Table of Contents
Report Sheet: Lab 7 - Electron Dot Structures and Molecular Shapes
This report details the findings and analysis from Lab 7, focusing on the construction of electron dot structures (Lewis structures) and the prediction of molecular shapes using the Valence Shell Electron Pair Repulsion (VSEPR) theory. Understanding these concepts is crucial for predicting the properties and reactivity of molecules.
Introduction
The objective of this lab was to develop proficiency in drawing electron dot structures for various molecules and ions, and to subsequently apply the VSEPR theory to predict their three-dimensional geometries. Electron dot structures visually represent the valence electrons in an atom or molecule, illustrating bonding and lone pairs. VSEPR theory, based on the electrostatic repulsion between electron pairs, allows us to predict the arrangement of atoms around a central atom, leading to specific molecular shapes. This report will cover the procedures followed, the observed data, and the analysis of the results, demonstrating the correlation between electron dot structures and molecular shapes.
Materials and Methods
The materials used in this lab were limited to pencils, erasers, and a periodic table. No specific chemicals or equipment were required, as the focus was on the theoretical application of electron dot structures and VSEPR theory. The methodology involved the following steps:
-
Determining Valence Electrons: For each molecule or ion assigned, we first determined the total number of valence electrons contributed by each atom. This involved referencing the group number of each element in the periodic table. For ions, we adjusted the electron count accordingly (adding electrons for negative ions, subtracting for positive ions).
-
Drawing Electron Dot Structures: We then constructed the electron dot structures, placing the least electronegative atom as the central atom (except hydrogen, which is always terminal). We aimed for each atom (except hydrogen) to achieve a complete octet (eight valence electrons), with exceptions for elements such as boron and beryllium. Multiple bonds (double or triple bonds) were introduced where necessary to satisfy the octet rule.
-
Identifying Electron Pair Geometry: Using the completed electron dot structures, we determined the electron pair geometry – the arrangement of all electron pairs (both bonding and lone pairs) around the central atom. This was based on the total number of electron pairs.
-
Predicting Molecular Geometry: Finally, we predicted the molecular geometry – the arrangement of only the atoms in the molecule, considering the presence of any lone pairs. The lone pairs influence the molecular shape, as they repel bonding pairs, causing distortions from idealized geometries.
Results and Discussion
The following tables summarize the results for several molecules and ions studied in the lab. Each entry includes the molecule or ion, its electron dot structure, the electron pair geometry, the molecular geometry, and a brief description of any observed deviations from idealized geometries.
Table 1: Simple Molecules
Molecule/Ion | Electron Dot Structure | Electron Pair Geometry | Molecular Geometry | Description |
---|---|---|---|---|
CH₄ (Methane) | Tetrahedral | Tetrahedral | Idealized tetrahedral shape with bond angles of approximately 109.5°. | |
H₂O (Water) | Tetrahedral | Bent | Two lone pairs on oxygen cause a bent shape with a bond angle less than 109.5° (approximately 104.5°). | |
NH₃ (Ammonia) | Tetrahedral | Trigonal Pyramidal | One lone pair on nitrogen causes a trigonal pyramidal shape with bond angles slightly less than 109.5°. | |
CO₂ (Carbon Dioxide) | O=C=O | Linear | Linear | All atoms are in a straight line. |
BF₃ (Boron Trifluoride) | Trigonal Planar | Trigonal Planar | Boron has only six valence electrons in this molecule, satisfying the exception to the octet rule. |
Table 2: Molecules with Multiple Bonds
Molecule/Ion | Electron Dot Structure | Electron Pair Geometry | Molecular Geometry | Description |
---|---|---|---|---|
C₂H₄ (Ethene) | Trigonal Planar | Trigonal Planar | The presence of a double bond doesn't affect the overall trigonal planar shape around each carbon atom. | |
C₂H₂ (Ethyne) | Linear | Linear | The triple bond results in a linear electron pair and molecular geometry. |
Table 3: Polyatomic Ions
Molecule/Ion | Electron Dot Structure | Electron Pair Geometry | Molecular Geometry | Description |
---|---|---|---|---|
SO₄²⁻ (Sulfate Ion) | Tetrahedral | Tetrahedral | Four oxygen atoms surround the sulfur atom with no lone pairs on the central atom, resulting in an ideal tetrahedral shape. | |
NO₃⁻ (Nitrate Ion) | Trigonal Planar | Trigonal Planar | The presence of resonance structures indicates electron delocalization, contributing to the planar geometry. | |
NH₄⁺ (Ammonium Ion) | Tetrahedral | Tetrahedral | The positive charge results from the loss of an electron from ammonia, but the shape remains tetrahedral. |
Conclusion
This lab provided valuable hands-on experience in applying fundamental concepts of chemical bonding and molecular geometry. By constructing electron dot structures and using VSEPR theory, we successfully predicted the three-dimensional shapes of various molecules and ions. The results demonstrate the strong correlation between the arrangement of electron pairs and the resulting molecular geometry. Understanding these concepts is crucial for predicting the physical and chemical properties of molecules, including their reactivity, polarity, and intermolecular forces.
The accuracy of VSEPR predictions relies on the assumption that electron pairs repel each other equally. However, deviations from idealized geometries can occur due to factors such as the presence of multiple bonds or different types of electron pairs. For example, the bond angle in water is less than the ideal tetrahedral angle (109.5°) due to the greater repulsive force of lone pairs compared to bonding pairs.
Further exploration could involve investigating more complex molecules with multiple central atoms or those exhibiting significant deviations from idealized geometries. Additionally, studying the impact of molecular shape on properties like polarity and reactivity would provide a more comprehensive understanding of the relationship between structure and function in molecules. The ability to accurately predict molecular geometry is a vital skill for chemists, enabling the prediction of a molecule's properties and its potential reactivity with other molecules. This lab provided a solid foundation for mastering these essential concepts.
Further Applications and Considerations
The principles learned in this lab extend far beyond simple molecule analysis. Understanding electron dot structures and molecular geometries is fundamental to:
-
Organic Chemistry: Predicting the shapes of organic molecules, including their conformations and isomers, is crucial for understanding their reactivity and biological function.
-
Inorganic Chemistry: The shapes of inorganic complexes influence their properties, such as color, magnetism, and reactivity.
-
Biochemistry: Understanding the three-dimensional structures of proteins and other biomolecules is essential for comprehending their function in biological systems. The shapes dictate how they interact with other molecules and perform their biological roles.
-
Material Science: The molecular geometries of materials dictate their physical and chemical properties, impacting their suitability for various applications, such as semiconductors and catalysts.
-
Spectroscopy: Molecular shapes significantly influence spectroscopic properties, such as infrared and Raman spectra, which can be used to identify and characterize molecules.
By mastering the principles covered in this lab, one gains a deeper appreciation for the intricate relationship between the molecular structure and its properties. This knowledge forms the basis for further explorations into the fascinating world of chemistry and its applications in various fields. The ability to visualize and predict molecular shapes is a key component of successful scientific investigation across numerous disciplines.
Latest Posts
Latest Posts
-
Estimating A Phase Transition Temperature From Standard Thermodynamic Data
May 10, 2025
-
What Is The Name Of The Covalent Compound So3
May 10, 2025
-
The Total Kinetic Energy Of Particles In A Substance
May 10, 2025
-
Calculate The Mass Percent Composition Of Nitrogen In Nh3
May 10, 2025
-
At 25 C The Equilibrium Partial Pressures For The Reaction
May 10, 2025
Related Post
Thank you for visiting our website which covers about Report Sheet Lab 7 Electron Dot Structures And Molecular Shape . We hope the information provided has been useful to you. Feel free to contact us if you have any questions or need further assistance. See you next time and don't miss to bookmark.