Secondary Structure Is Characterized By Which Type Of Interactions
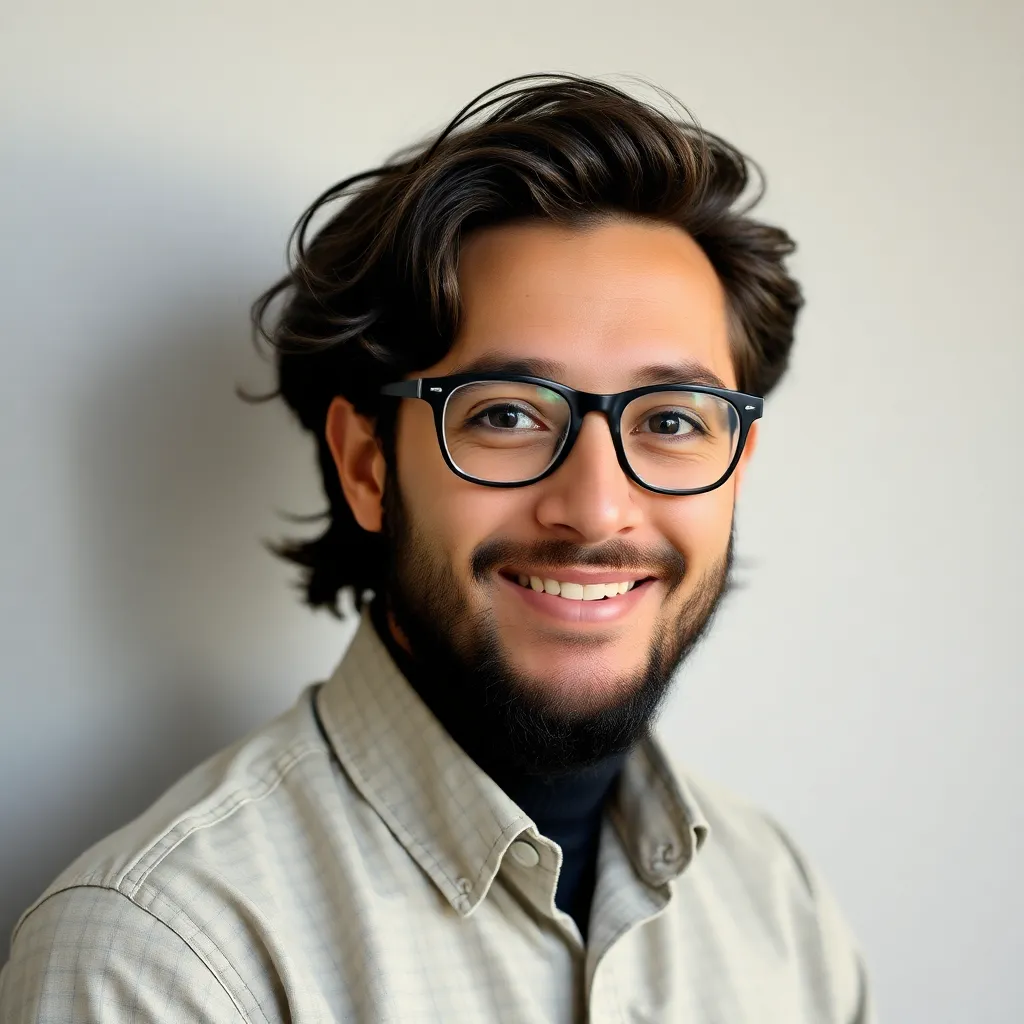
Muz Play
May 11, 2025 · 6 min read

Table of Contents
Secondary Structure is Characterized by Which Type of Interactions?
Secondary structure in proteins and nucleic acids represents a crucial level of organization, bridging the gap between the primary sequence (the linear order of amino acids or nucleotides) and the complex three-dimensional tertiary structure. Understanding the types of interactions driving secondary structure formation is fundamental to comprehending protein function and stability, as well as the behavior of nucleic acids like DNA and RNA. This article will delve into the specific interactions responsible for the formation and maintenance of secondary structures in both proteins and nucleic acids.
Secondary Structure in Proteins: The Reign of Hydrogen Bonds
Protein secondary structure primarily arises from hydrogen bonds between the backbone atoms of the polypeptide chain. These bonds are non-covalent interactions, relatively weak individually, but collectively exert a strong influence on the protein's conformation. The most common secondary structures – alpha-helices and beta-sheets – are directly attributable to these hydrogen bonding patterns.
Alpha-Helices: A Spiral Staircase of Hydrogen Bonds
The alpha-helix is a right-handed coiled conformation stabilized by hydrogen bonds between the carbonyl oxygen of one amino acid residue and the amide hydrogen of the amino acid four residues down the chain (n and n+4). This creates a regular repeating pattern of hydrogen bonds that runs parallel to the helix axis, reinforcing its stability. The R-groups (side chains) of the amino acids extend outwards from the helix core, minimizing steric clashes and influencing helix propensity.
Factors affecting alpha-helix stability:
- Amino acid composition: Certain amino acids, like proline (due to its rigid cyclic structure) and glycine (due to its flexibility), disrupt alpha-helix formation. Conversely, amino acids with long hydrophobic side chains often favor alpha-helix formation.
- Charge distribution: Clusters of charged residues can destabilize the helix by electrostatic repulsion.
- Solvent accessibility: The solvent environment can also influence helix stability.
Beta-Sheets: Extended Strands Linked by Hydrogen Bonds
Beta-sheets consist of extended polypeptide chains arranged side-by-side to form a pleated sheet structure. These strands are held together by hydrogen bonds between the carbonyl oxygen of one strand and the amide hydrogen of an adjacent strand. Beta-sheets can be parallel (strands running in the same direction) or antiparallel (strands running in opposite directions). Antiparallel beta-sheets generally exhibit stronger hydrogen bonds due to the linear alignment of the hydrogen bond donors and acceptors.
Variations in Beta-Sheets:
- Parallel beta-sheets: Hydrogen bonds are slightly weaker and less linear compared to antiparallel sheets.
- Antiparallel beta-sheets: Hydrogen bonds are stronger and more linear.
- Mixed beta-sheets: These contain both parallel and antiparallel sections.
Loops and Turns: Connecting the Secondary Structure Elements
In addition to alpha-helices and beta-sheets, proteins also contain loops and turns that connect these secondary structure elements. These regions are often less ordered but still play crucial roles in protein folding and function. Hydrogen bonds, along with other non-covalent interactions like van der Waals forces and hydrophobic interactions, contribute to the stability of loops and turns.
Secondary Structure in Nucleic Acids: A Double Helix of Hydrogen Bonds and Base Stacking
The secondary structure of nucleic acids, particularly DNA and RNA, is largely determined by hydrogen bonding between complementary base pairs and base stacking interactions.
DNA Double Helix: A Masterpiece of Hydrogen Bonding and Base Stacking
The iconic double helix of DNA is stabilized by hydrogen bonds between complementary base pairs: adenine (A) with thymine (T) and guanine (G) with cytosine (C). A-T pairs form two hydrogen bonds, while G-C pairs form three, contributing to the higher stability of G-C rich DNA regions. Furthermore, base stacking interactions between adjacent bases contribute significantly to the stability of the double helix. These hydrophobic interactions minimize contact of the bases with water, favoring the stacked conformation.
Factors Influencing DNA Double Helix Stability:
- Base composition: G-C rich regions are more stable due to the presence of three hydrogen bonds per base pair.
- Ionic strength: Salt concentration influences the electrostatic interactions between the negatively charged phosphate backbone.
- Temperature: Higher temperatures disrupt hydrogen bonds and base stacking, leading to DNA denaturation (melting).
RNA Secondary Structure: A Diverse Array of Hydrogen Bonds and Base Stacking
RNA secondary structure is far more diverse than DNA's double helix. It includes stem-loops (hairpins), internal loops, bulges, and multi-branched junctions. All these structures are formed through hydrogen bonding between complementary bases, much like in DNA. However, RNA also forms non-canonical base pairs (i.e., not A-U or G-C), contributing to the structural complexity and functional versatility of RNA molecules. Base stacking remains crucial, particularly in helical regions.
RNA Secondary Structure Motifs:
- Stem-loops (hairpins): Formed by a stretch of complementary sequence folding back on itself, creating a double-helical stem and a single-stranded loop.
- Internal loops: Unpaired nucleotides within a double-helical region.
- Bulges: Unpaired nucleotides on one strand of a double-helical region.
- Multi-branched junctions: Points where multiple double-helical stems converge.
Beyond Hydrogen Bonds: Other Interactions Supporting Secondary Structure
While hydrogen bonds are the primary driving force behind protein and nucleic acid secondary structure, other interactions play important supporting roles:
- Hydrophobic interactions: The tendency of nonpolar side chains in proteins to cluster together, away from water, contributes to the overall stability of the folded structure. Base stacking in nucleic acids is also a type of hydrophobic interaction.
- Van der Waals forces: Weak, short-range attractive forces between atoms contribute to the overall packing and stability of the structure.
- Electrostatic interactions: Interactions between charged groups, including salt bridges and dipole-dipole interactions, can stabilize or destabilize secondary structures, depending on their arrangement.
The Importance of Secondary Structure in Biological Function
The secondary structure of proteins and nucleic acids is not just a structural feature; it's crucial for their biological function.
Proteins: Secondary structures form the basis for the higher-order tertiary and quaternary structures. The specific arrangement of alpha-helices and beta-sheets determines the protein's overall shape and functionality. For example, alpha-helices often participate in protein-protein interactions, while beta-sheets are frequently involved in forming stable structural cores.
Nucleic Acids: The secondary structure of RNA, in particular, is critical for its diverse functions, including catalysis (ribozymes), gene regulation, and protein synthesis. The specific folds of RNA molecules often dictate their interactions with other molecules, determining their biological role.
Conclusion: A Delicate Balance of Interactions
In conclusion, the secondary structure of proteins and nucleic acids is a fascinating consequence of a delicate balance of non-covalent interactions, with hydrogen bonding as the primary driving force. The specific patterns of hydrogen bonds, along with the contributions of hydrophobic interactions, van der Waals forces, and electrostatic interactions, dictate the formation of alpha-helices, beta-sheets, and the diverse range of nucleic acid secondary structures. These structures, in turn, lay the foundation for the complex three-dimensional architectures and ultimately the biological functions of proteins and nucleic acids. Understanding these interactions is therefore essential for comprehending the intricacies of life itself. Further research continues to unveil the subtleties of these interactions and their crucial roles in cellular processes.
Latest Posts
Latest Posts
-
Are Molecules The Same As Particles
May 12, 2025
-
Which Has A Larger Atomic Radius
May 12, 2025
-
Que Es Mas Largo Una Milla O Un Kilometro
May 12, 2025
-
How Are Mixture And Solution Alike
May 12, 2025
-
Gramatica B The Verb Gustar Answer Key
May 12, 2025
Related Post
Thank you for visiting our website which covers about Secondary Structure Is Characterized By Which Type Of Interactions . We hope the information provided has been useful to you. Feel free to contact us if you have any questions or need further assistance. See you next time and don't miss to bookmark.