Secondary Structures Are Stabilized By Which Type Of Interaction
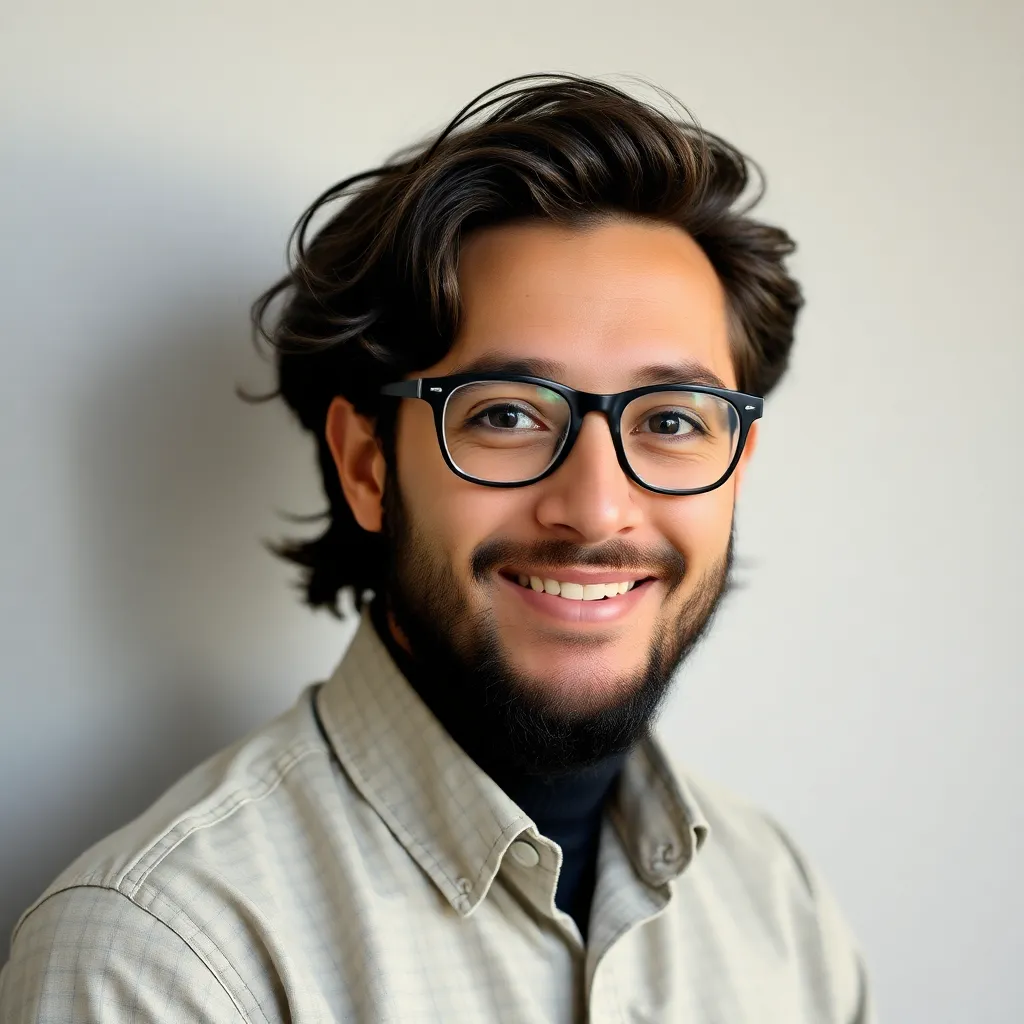
Muz Play
Apr 17, 2025 · 6 min read

Table of Contents
Secondary Structures are Stabilized by Which Type of Interaction?
Secondary structures in proteins and nucleic acids represent the intermediate level of organization between the primary sequence and the complex three-dimensional tertiary structure. These structures, including alpha-helices, beta-sheets, and turns in proteins, and stem-loops, hairpins, and bulges in nucleic acids, are fundamentally stabilized by non-covalent interactions. While the primary structure is dictated by the covalent peptide bonds (proteins) or phosphodiester bonds (nucleic acids), the formation and stability of secondary structures rely on a delicate balance of weaker forces. Understanding these interactions is crucial to comprehending protein folding, nucleic acid structure, and ultimately, biological function.
The Key Players: Non-Covalent Interactions
The stability of secondary structures hinges on several types of non-covalent interactions, each contributing to the overall free energy of the folded structure. These interactions are individually weak, but their cumulative effect, especially within a densely packed structure, can be remarkably strong. Let's explore the major players:
1. Hydrogen Bonds: The Backbone of Secondary Structure
Hydrogen bonds are arguably the most significant contributors to the stability of secondary structures. These bonds form between a hydrogen atom covalently bound to an electronegative atom (like oxygen or nitrogen) and another electronegative atom with a lone pair of electrons. In proteins, the hydrogen bonds are primarily formed between the backbone amide (-NH) and carbonyl (C=O) groups of amino acids.
-
Alpha-helices: In alpha-helices, hydrogen bonds form between the carbonyl oxygen of an amino acid residue and the amide hydrogen of the amino acid four residues down the chain. This creates a stable, rod-like structure with a characteristic 3.6 amino acid residues per turn. The consistent pattern of hydrogen bonds ensures the structural integrity of the helix.
-
Beta-sheets: Beta-sheets are formed by hydrogen bonds between adjacent polypeptide chains (or segments of the same chain) running in either parallel or antiparallel orientations. The hydrogen bonds are formed between the backbone amide and carbonyl groups of the adjacent strands. Antiparallel beta-sheets, where the strands run in opposite directions, generally exhibit stronger hydrogen bonds due to a more linear arrangement of the donor and acceptor atoms.
-
Nucleic Acids: In nucleic acids (DNA and RNA), hydrogen bonds are crucial for base pairing. Adenine (A) forms two hydrogen bonds with thymine (T) in DNA or uracil (U) in RNA, while guanine (G) forms three hydrogen bonds with cytosine (C). These hydrogen bonds are essential for the formation of the double helix structure in DNA and the various secondary structures in RNA, such as stem-loops and hairpins. The strength of these base pairs significantly influences the melting temperature of the nucleic acid.
2. Hydrophobic Interactions: The Driving Force
Hydrophobic interactions play a crucial, albeit indirect, role in stabilizing secondary structures. These interactions arise from the tendency of nonpolar amino acid side chains (e.g., leucine, valine, phenylalanine) to cluster together in an aqueous environment, minimizing their contact with water. This clustering effect is not a direct interaction between the hydrophobic groups themselves but rather a consequence of the increased entropy of water molecules when hydrophobic groups are excluded from the solvent.
-
Burying Hydrophobic Residues: In alpha-helices and beta-sheets, hydrophobic residues are often located in the interior of the structure, shielded from water. This burying of hydrophobic residues is a significant driving force for secondary structure formation because it increases the overall entropy of the system.
-
Nucleic Acid Stability: While less pronounced than in proteins, hydrophobic interactions also play a role in the stability of nucleic acid secondary structures. Base stacking, where adjacent bases interact through van der Waals forces and hydrophobic interactions, contributes to the stability of the double helix and other secondary structures.
3. Van der Waals Forces: Weak but Numerous
Van der Waals forces are weak, short-range attractions between molecules or parts of molecules. They arise from temporary fluctuations in electron distribution, creating transient dipoles that induce dipoles in neighboring atoms or molecules. While individually weak, the cumulative effect of numerous van der Waals interactions within a densely packed secondary structure is substantial.
-
Close Packing: The close packing of atoms in secondary structures maximizes the number of van der Waals interactions, contributing to the overall stability of the structure.
-
Base Stacking: In nucleic acids, van der Waals forces are particularly important in base stacking, contributing significantly to the stability of the double helix. The planar aromatic bases are stacked on top of each other, maximizing van der Waals interactions.
4. Electrostatic Interactions: Salt Bridges and More
Electrostatic interactions, involving attractions between oppositely charged groups, can also influence the stability of secondary structures. These interactions are particularly important when charged amino acid side chains are involved.
-
Salt Bridges: Salt bridges, formed between positively and negatively charged amino acid side chains, can contribute to the stability of proteins, especially when these charged groups are located on the surface of the structure. However, the strength of these interactions is heavily dependent on the surrounding environment and the proximity of the charged residues.
-
Phosphate Backbone: The negatively charged phosphate backbone in nucleic acids influences the overall stability of the structure through electrostatic interactions with surrounding ions and water molecules. These interactions can influence the conformation and stability of the nucleic acid secondary structures.
5. Conformational Entropy: A Balancing Act
The formation of a secondary structure involves a decrease in conformational entropy. This is because the flexible polypeptide chain or nucleic acid strand becomes more ordered. To counteract this entropic penalty, the system must gain an energetically favorable amount of enthalpy through the non-covalent interactions discussed above. The balance between the enthalpy gained and the entropy lost determines whether a secondary structure will form and its stability.
Factors Affecting Secondary Structure Stability
Several factors beyond the types of interactions discussed above can influence the stability of secondary structures:
-
Amino Acid Sequence (Proteins): The sequence of amino acids dictates the propensity of a polypeptide chain to adopt certain secondary structures. Some amino acids, like proline, are known to disrupt alpha-helices due to their rigid cyclic structure. Others, like glycine, are highly flexible and can readily adopt various conformations.
-
Solvent Conditions (Proteins & Nucleic Acids): The surrounding environment, including pH, temperature, and ionic strength, significantly impacts the stability of secondary structures. Changes in these parameters can disrupt non-covalent interactions and lead to unfolding or conformational changes.
-
Sequence-Dependent Interactions (Nucleic Acids): The specific sequence of bases in nucleic acids plays a significant role in determining the secondary structure. The relative abundance of G-C versus A-T (or A-U) base pairs, which differ in the number of hydrogen bonds, impacts the melting temperature and overall stability.
Conclusion: A Complex interplay
The stability of secondary structures in proteins and nucleic acids is a consequence of a complex interplay between several types of non-covalent interactions. While hydrogen bonds are arguably the most significant contributors, hydrophobic interactions, van der Waals forces, electrostatic interactions, and the balance between enthalpy and entropy all play critical roles. Understanding these interactions is crucial for comprehending protein folding, nucleic acid structure, function, and the design of novel biomolecules. Further research continues to refine our understanding of the subtle nuances of these forces and their impact on biological systems. This detailed understanding is crucial in fields ranging from drug discovery to nanotechnology, where manipulating protein and nucleic acid structures is paramount. The intricate balance of forces underpinning these essential structural elements highlights the elegance and complexity of biological systems. Future advancements in our understanding of these interactions will undoubtedly lead to exciting breakthroughs in various scientific disciplines.
Latest Posts
Latest Posts
-
How To Calculate Uncertainty Of Measurement
Apr 19, 2025
-
Line Integral With Respect To Arc Length
Apr 19, 2025
-
What Four Bases Are Found In Rna
Apr 19, 2025
-
What Is Beyonces Song Lemonade About
Apr 19, 2025
-
Give The Major Force Between Ethanol And Water
Apr 19, 2025
Related Post
Thank you for visiting our website which covers about Secondary Structures Are Stabilized By Which Type Of Interaction . We hope the information provided has been useful to you. Feel free to contact us if you have any questions or need further assistance. See you next time and don't miss to bookmark.