Temperature Change In Adiabatic Heating Occurs Due To
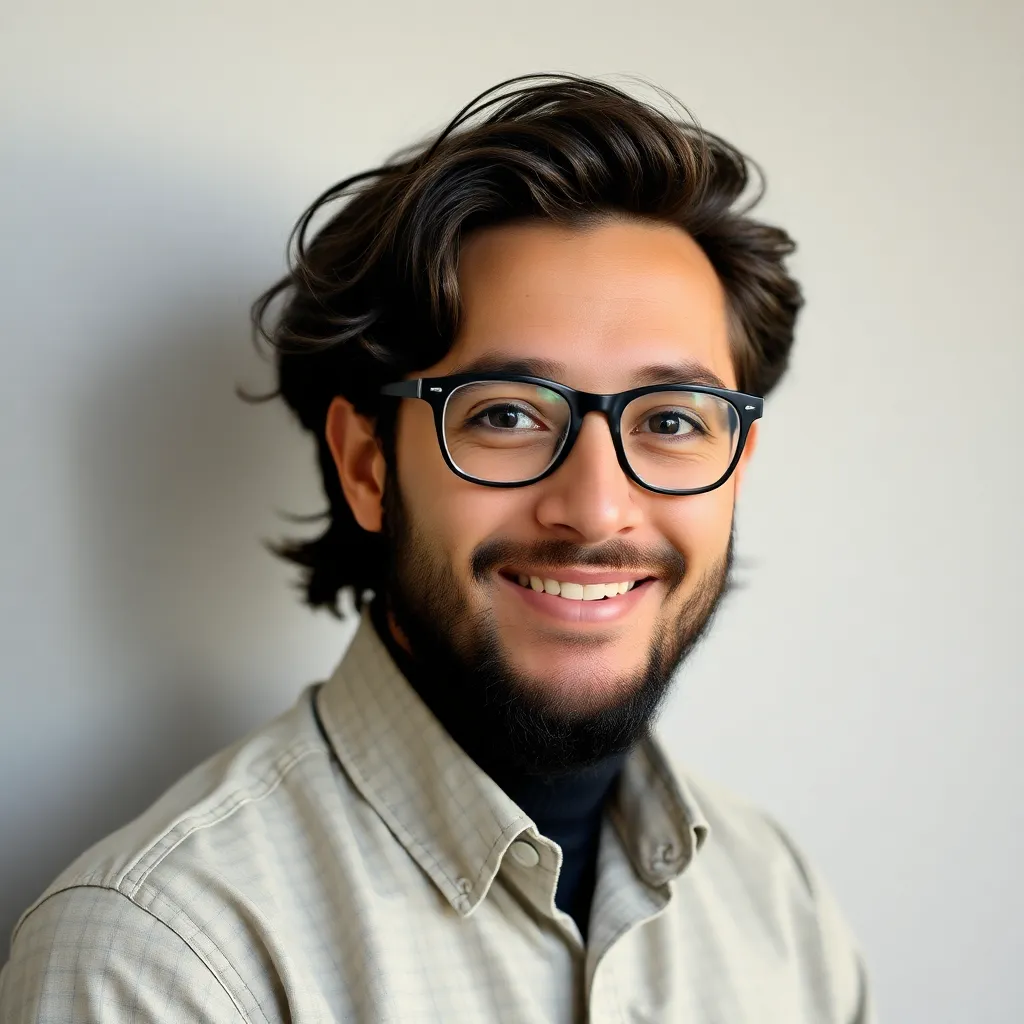
Muz Play
May 11, 2025 · 5 min read
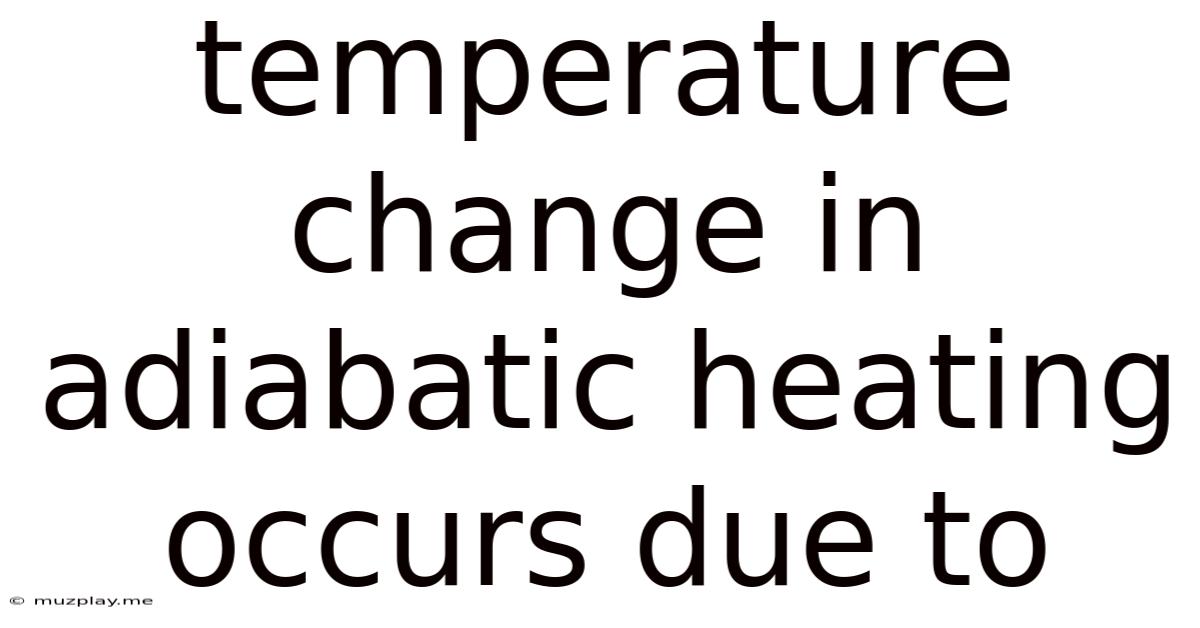
Table of Contents
Temperature Change in Adiabatic Heating: A Deep Dive
Adiabatic heating, a process fundamental to meteorology and thermodynamics, describes the temperature rise of a gas due to compression without heat exchange with its surroundings. Understanding this phenomenon is crucial for explaining various atmospheric processes, from the formation of clouds to the dynamics of weather systems. This comprehensive article delves deep into the mechanics of adiabatic heating, exploring the underlying physics, its applications, and associated factors that influence the magnitude of temperature change.
The Physics Behind Adiabatic Heating: Compression and Internal Energy
The core principle governing adiabatic heating lies in the first law of thermodynamics: the change in internal energy (ΔU) of a system equals the heat added (Q) minus the work done (W) by the system. Mathematically, this is expressed as ΔU = Q - W.
In an adiabatic process, by definition, there's no heat exchange with the environment (Q = 0). Therefore, the equation simplifies to ΔU = -W. When a gas is compressed adiabatically, work is done on the gas (W is negative), resulting in an increase in its internal energy (ΔU is positive). This increase in internal energy manifests as a rise in temperature. Conversely, adiabatic expansion leads to cooling as the gas does work on its surroundings, decreasing its internal energy.
The Role of Pressure and Volume
The relationship between pressure (P), volume (V), and temperature (T) in an adiabatic process is governed by the adiabatic equation:
PV<sup>γ</sup> = constant
where γ (gamma) is the adiabatic index (ratio of specific heats), representing the ratio of the gas's specific heat at constant pressure (Cp) to its specific heat at constant volume (Cv). The value of γ depends on the composition of the gas; for dry air, γ is approximately 1.4.
This equation highlights the crucial role of pressure and volume changes in driving temperature variations during adiabatic processes. As pressure increases (e.g., due to compression), the volume decreases, leading to a rise in temperature, and vice versa.
Adiabatic Lapse Rate: A Key Concept
The adiabatic lapse rate is a crucial concept for understanding temperature changes in the atmosphere. It represents the rate at which the temperature of a parcel of air changes as it rises or sinks adiabatically. There are two primary adiabatic lapse rates:
1. Dry Adiabatic Lapse Rate (DALR):
This applies to unsaturated air (air that hasn't reached its dew point). The DALR is approximately 9.8 °C per 1000 meters (or 5.4 °F per 1000 feet). As an unsaturated air parcel rises, it expands due to decreasing atmospheric pressure, leading to adiabatic cooling at this rate. Conversely, sinking air compresses and heats at the same rate.
2. Moist Adiabatic Lapse Rate (MALR):
This applies to saturated air (air that has reached its dew point and contains water vapor). The MALR is significantly less than the DALR, typically ranging from 4 °C to 7 °C per 1000 meters (or 2.2 °F to 3.8 °F per 1000 feet). The lower rate is due to the release of latent heat during condensation. As saturated air rises, condensation occurs, releasing latent heat, which partially offsets the cooling effect of expansion.
Factors Influencing Adiabatic Heating Magnitude
Several factors influence the magnitude of temperature change during adiabatic heating:
1. Initial Temperature and Pressure:
The initial temperature and pressure of the gas significantly impact the final temperature after adiabatic compression. Higher initial temperatures and pressures generally result in larger temperature increases after compression.
2. Degree of Compression:
The extent of compression directly affects the temperature change. Greater compression leads to a more substantial temperature increase. This is reflected in the adiabatic equation; a larger decrease in volume corresponds to a larger increase in temperature for a given γ.
3. Composition of the Gas:
The adiabatic index (γ) depends on the gas's composition. Gases with different compositions will exhibit different temperature changes under the same adiabatic compression conditions. The presence of water vapor, for example, reduces the lapse rate due to latent heat release.
4. Rate of Compression:
The speed of compression can also play a role, particularly in situations where the process is not perfectly adiabatic. Very rapid compression can lead to deviations from true adiabatic behavior due to heat transfer.
Applications of Adiabatic Heating in Meteorology and Beyond
Adiabatic heating plays a vital role in numerous atmospheric phenomena:
1. Cloud Formation:
As air rises adiabatically, it cools, leading to condensation and cloud formation. Understanding adiabatic lapse rates is crucial for predicting cloud development and precipitation.
2. Mountain Waves and Rotor Clouds:
Air forced over mountain ranges undergoes adiabatic compression and expansion, leading to the formation of lee waves and lenticular clouds. The adiabatic processes are central to the dynamics of these spectacular weather phenomena.
3. Chinook Winds:
Chinook winds are warm, dry winds that descend mountain slopes. Adiabatic compression during descent leads to significant warming, resulting in rapid temperature increases downwind.
4. Atmospheric Stability:
The comparison between the environmental lapse rate (the actual temperature decrease with altitude) and the adiabatic lapse rates helps determine atmospheric stability. If the environmental lapse rate is greater than the adiabatic lapse rate, the atmosphere is unstable, favoring convection and vertical air motion.
Beyond Meteorology: Applications in Other Fields
The principles of adiabatic heating are not limited to meteorology. They find applications in various fields, including:
1. Internal Combustion Engines:
The compression stroke in internal combustion engines involves adiabatic compression of the air-fuel mixture, leading to a significant temperature rise that initiates combustion.
1. Refrigeration and Air Conditioning:
Adiabatic expansion is utilized in refrigeration cycles. The expansion of a refrigerant gas causes adiabatic cooling, creating a low-temperature environment for heat absorption.
Conclusion: A Fundamental Process with Broad Implications
Adiabatic heating, driven by the compression of a gas without heat exchange, is a fundamental thermodynamic process with far-reaching consequences. Understanding the physics behind adiabatic heating, the adiabatic lapse rates, and the factors influencing temperature changes is crucial for interpreting various natural phenomena, from cloud formation to wind patterns. Its applications extend beyond meteorology into engineering and other scientific domains, solidifying its importance as a cornerstone of thermodynamics and atmospheric science. Further research continues to refine our understanding of this crucial process and its role in shaping our climate and weather systems. The intricacies of adiabatic processes continue to inspire investigations into their impact on various aspects of our environment and technology. The ongoing exploration of this field promises further advancements in our ability to model, predict, and ultimately understand the complex interactions governing the physical world.
Latest Posts
Latest Posts
-
Select All The Characteristics Of Life
May 11, 2025
-
Multiunit Smooth Muscle Cells Are Stimulated
May 11, 2025
-
Determine Whether 2 Chloro 3 Methylbutane Contains A Chiral Center
May 11, 2025
-
Figure 27 2 Surface Features Of The Heart
May 11, 2025
-
Is Oil Polar Or Non Polar
May 11, 2025
Related Post
Thank you for visiting our website which covers about Temperature Change In Adiabatic Heating Occurs Due To . We hope the information provided has been useful to you. Feel free to contact us if you have any questions or need further assistance. See you next time and don't miss to bookmark.