The Attraction Of A Metallic Cation For Delocalized Electrons
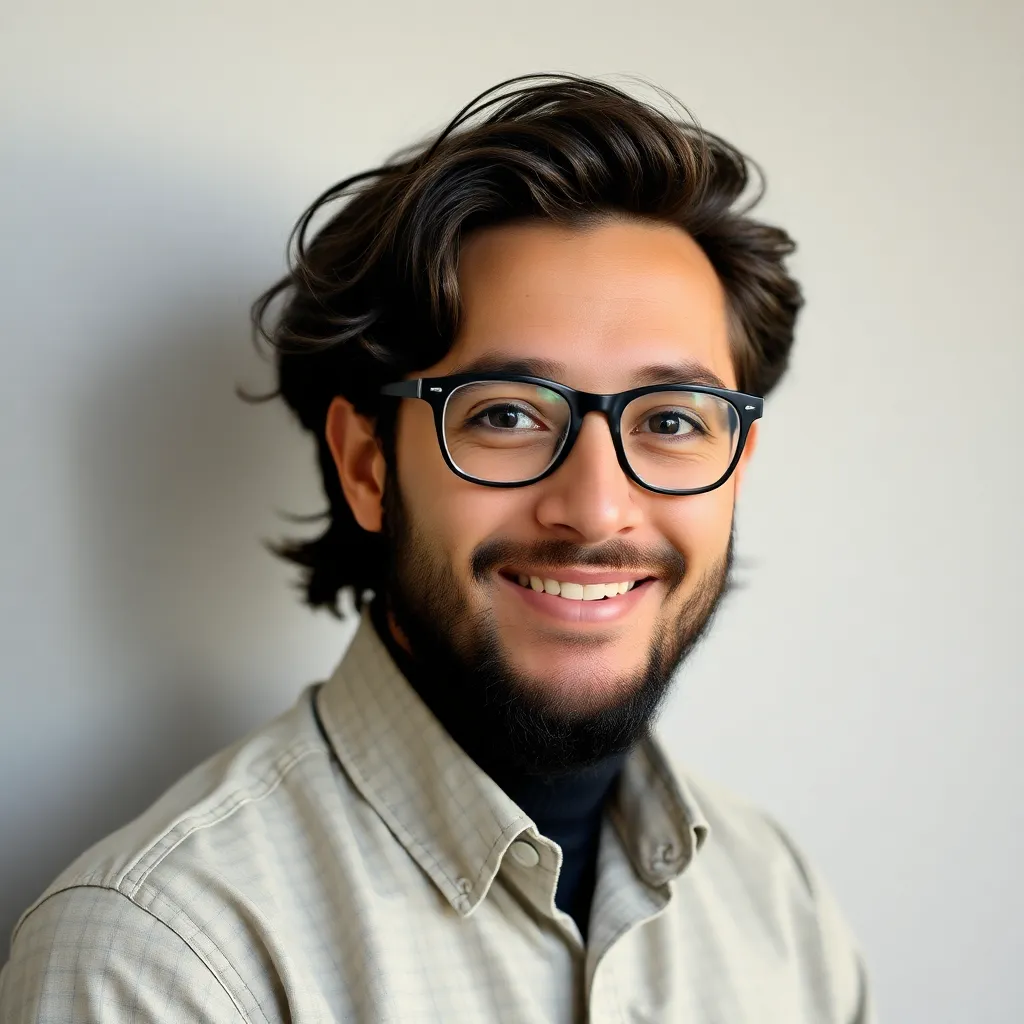
Muz Play
May 09, 2025 · 6 min read
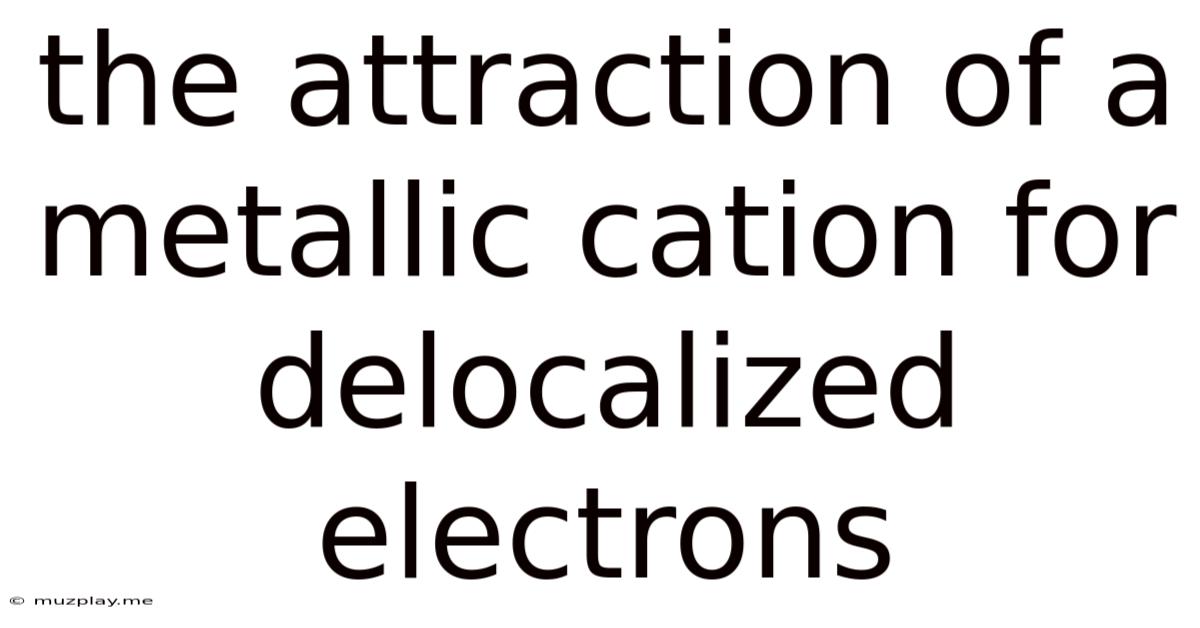
Table of Contents
The Allure of the Metal: Exploring the Attraction Between Metallic Cations and Delocalized Electrons
The shimmering beauty of metals, their malleability, and excellent conductivity are all consequences of a fundamental interaction: the strong attraction between metallic cations and delocalized electrons. This seemingly simple interaction is a cornerstone of materials science, influencing a vast array of properties and underpinning the behavior of countless metallic materials. This article delves deep into the nature of this attraction, exploring its origins, consequences, and implications across various applications.
Understanding the Players: Metallic Cations and Delocalized Electrons
Before examining the interaction itself, let's define the key players. Metallic cations are positively charged metal ions. When a metal atom loses one or more valence electrons, it becomes a cation. This electron loss is a defining characteristic of metals and is driven by their relatively low ionization energies. The electrons aren't tightly bound to individual atoms, leading to the next crucial component: delocalized electrons. These are valence electrons that are not associated with any particular atom but are free to move throughout the entire metallic lattice. This "sea" or "cloud" of delocalized electrons is what distinguishes metals from other materials.
The Metallic Bond: A Sea of Electrons
The attraction between metallic cations and delocalized electrons constitutes the metallic bond. Unlike ionic or covalent bonds, the metallic bond is non-directional; the electrons are not localized between specific atoms but rather spread throughout the entire metal structure. This is crucial to understanding the unique properties of metals. The delocalized electrons act as a "glue," holding the positively charged cations together in a stable, three-dimensional lattice. The strength of this bond depends on several factors, including:
- Charge density of the cation: Higher charge density (smaller cation with larger positive charge) leads to stronger attraction to the electron cloud.
- Number of delocalized electrons: More delocalized electrons contribute to a denser electron cloud and stronger bonding.
- Size of the cation: Smaller cations result in stronger attraction due to reduced electron-cation distance.
The Electrostatic Dance: Nature of the Attraction
At the heart of the metallic bond lies electrostatic attraction. The positively charged cations are strongly attracted to the negatively charged delocalized electrons. This attraction is not a localized bond like in covalent compounds but rather a collective interaction across the entire metal structure. Imagine a lattice of positive charges immersed in a sea of negative charges – the electrostatic forces create a cohesive and stable structure. This is why metals are typically solid at room temperature, except for mercury, which has weaker metallic bonding.
Beyond Simple Electrostatics: Quantum Mechanical Perspective
While the electrostatic model provides a good basic understanding, a complete description requires a quantum mechanical perspective. The delocalized electrons are not simply static particles; they occupy molecular orbitals that extend throughout the entire metal lattice. These molecular orbitals are formed by the overlap of atomic orbitals from numerous metal atoms. The electrons are not confined to specific energy levels, rather, they exist in a continuous band of energy levels known as the valence band. The higher the number of delocalized electrons, the broader and more populated the valence band becomes.
The energy levels within the valence band are extremely closely spaced. This near-continuity of energy levels allows electrons to move freely under the influence of an applied electric field, explaining the high electrical conductivity of metals. The ease with which electrons move through these delocalized orbitals also explains the excellent thermal conductivity of metals, as thermal energy is efficiently transferred through the movement of these charge carriers.
Consequences of the Attraction: Properties of Metals
The unique attraction between metallic cations and delocalized electrons leads to a suite of characteristic metallic properties:
1. Electrical Conductivity:
The free movement of delocalized electrons allows metals to conduct electricity efficiently. When an electric field is applied, the electrons flow readily, carrying the electrical current. This property is crucial in countless applications, from electrical wiring to electronics. The higher the electron density and the greater the mobility of the electrons, the higher the electrical conductivity.
2. Thermal Conductivity:
Similar to electrical conductivity, the delocalized electrons also contribute to excellent thermal conductivity. Thermal energy is readily transferred through the movement of these electrons, allowing metals to efficiently conduct heat. This is why metals are often used in applications involving heat transfer, such as cooking utensils and heat sinks.
3. Malleability and Ductility:
The non-directional nature of the metallic bond allows metal atoms to slide past each other without breaking the bonds. This leads to the characteristic malleability (ability to be hammered into sheets) and ductility (ability to be drawn into wires) of metals. The sea of delocalized electrons acts as a buffer, preventing the repulsive forces between cations from causing the structure to shatter under deformation.
4. Metallic Luster:
The interaction of light with the delocalized electrons gives metals their characteristic metallic luster. The electrons absorb and re-emit light across a wide range of frequencies, resulting in the shiny reflective surface of most metals. The surface smoothness also contributes to this reflection.
5. Hardness and Strength:
While malleable and ductile, metals can also exhibit significant hardness and strength. These properties are influenced by the strength of the metallic bond, the arrangement of the metal atoms in the lattice, and the presence of impurities or alloying elements.
Beyond Pure Metals: Alloys and Their Behavior
The properties of metals can be significantly modified by alloying – the addition of other elements. Alloying alters the electron density, affects the lattice structure, and can introduce new interactions, thereby influencing the overall strength, hardness, conductivity, and other properties of the material. For example, steel, an alloy of iron and carbon, is significantly stronger and harder than pure iron. The carbon atoms interact with the iron lattice and delocalized electrons, strengthening the overall structure.
Applications: A World Shaped by Metallic Bonds
The unique properties stemming from the attraction between metallic cations and delocalized electrons are essential in a vast range of applications across multiple fields:
-
Construction: Steel, aluminum, and various alloys form the backbone of modern construction, providing structural integrity and strength.
-
Transportation: The lightweight strength of aluminum alloys makes them ideal for aircraft construction. Steel is ubiquitous in automobiles and trains.
-
Electronics: Copper and other metals are vital for electrical wiring and components in electronic devices.
-
Medical Implants: Biocompatible metals and alloys are used in medical implants, taking advantage of their strength, biocompatibility and corrosion resistance.
-
Catalysis: Metals and metal oxides act as catalysts in many chemical processes, facilitating reactions by providing surfaces for the adsorption and reaction of molecules.
Conclusion: A Fundamental Interaction with Profound Consequences
The seemingly simple attraction between metallic cations and delocalized electrons is a cornerstone of materials science, governing the properties and applications of a vast array of metallic materials. Understanding the intricacies of this interaction – from the basic electrostatic forces to the complex quantum mechanical descriptions – is critical to designing and developing new materials with tailored properties for diverse technological applications. The ongoing research in materials science continues to reveal new facets of this fundamental interaction, pushing the boundaries of our understanding and leading to further innovations in materials technology. The allure of the metal remains a powerful force driving technological advancement.
Latest Posts
Latest Posts
-
How Is A Buyers Responsiveness To Price Changes Measured
May 10, 2025
-
As Cell Size Goes Effectiveness Of Diffusion And Osmosis Goes
May 10, 2025
-
6 Steps Of Protein Synthesis In The Correct Order
May 10, 2025
-
Is Copper A Nonmetal Metal Or Metalloid
May 10, 2025
-
Which Is A Homologous Structure To The Human Forearm
May 10, 2025
Related Post
Thank you for visiting our website which covers about The Attraction Of A Metallic Cation For Delocalized Electrons . We hope the information provided has been useful to you. Feel free to contact us if you have any questions or need further assistance. See you next time and don't miss to bookmark.