The Basic Unit Of Heredity Is The
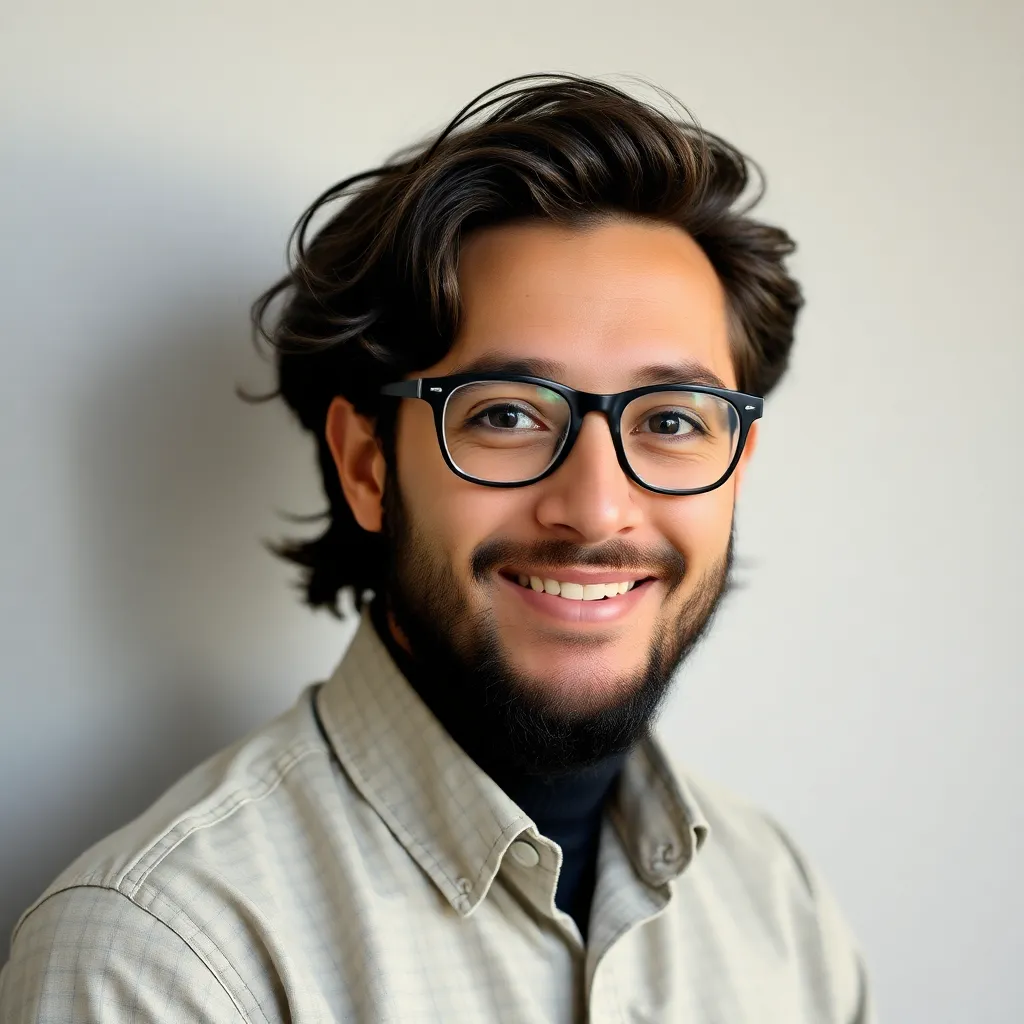
Muz Play
Apr 24, 2025 · 8 min read

Table of Contents
The Basic Unit of Heredity is the Gene: A Deep Dive into Genetics
The fundamental unit of heredity, the very essence of what makes us who we are, is the gene. This seemingly simple word encompasses a complex world of biological mechanisms, influencing everything from our eye color to our susceptibility to certain diseases. Understanding genes is key to understanding life itself. This comprehensive article will explore the gene in detail, delving into its structure, function, and the broader implications of genetic inheritance.
What is a Gene?
At its most basic level, a gene is a specific sequence of DNA (deoxyribonucleic acid) that provides the instructions for building a particular protein or functional RNA molecule. These proteins and RNA molecules are the workhorses of the cell, carrying out a vast array of functions necessary for life. Genes are not simply static blueprints; they are dynamic entities, subject to regulation and modification throughout an organism's life.
Think of DNA as a vast library containing countless books (genes). Each book contains specific instructions (genetic code) to build a particular protein (or RNA molecule). The order of the "letters" (nucleotides: adenine, guanine, cytosine, and thymine) within the gene dictates the sequence of amino acids that form the protein. This protein then performs its specific role within the cell and contributes to the overall phenotype (observable characteristics) of the organism.
The Structure of a Gene
A typical gene consists of several key components:
- Promoter Region: This region acts as a "switch," controlling when and how much of the gene is expressed (transcribed into RNA). Transcription factors, specialized proteins, bind to the promoter to initiate the process of gene expression.
- Coding Sequence (Exons): This section of the gene contains the actual instructions for building the protein. The sequence of nucleotides in the exons determines the amino acid sequence of the protein.
- Non-coding Sequences (Introns): These are regions within the gene that do not code for protein. Initially, they are transcribed into RNA along with the exons, but they are subsequently removed through a process called splicing before the RNA is translated into a protein. Introns play a surprisingly complex role in gene regulation and expression.
- Terminator Region: This signals the end of the gene and terminates the transcription process.
The specific arrangement and sequence of these components vary widely among genes, reflecting the diversity of their functions.
Gene Expression: From DNA to Protein
The process of turning a gene "on" and producing its corresponding protein or RNA is known as gene expression. This is a tightly regulated process, ensuring that the right proteins are produced at the right time and in the right amounts. Gene expression involves two major steps:
1. Transcription: DNA to RNA
Transcription is the process of copying the DNA sequence of a gene into a complementary molecule called messenger RNA (mRNA). This occurs in the nucleus of the cell with the help of an enzyme called RNA polymerase. RNA polymerase binds to the promoter region of the gene, unwinds the DNA double helix, and synthesizes an mRNA molecule that is complementary to the DNA template strand.
2. Translation: RNA to Protein
Translation is the process of converting the mRNA sequence into a protein. This takes place in the cytoplasm of the cell, on structures called ribosomes. Ribosomes "read" the mRNA sequence in groups of three nucleotides called codons. Each codon specifies a particular amino acid. Transfer RNA (tRNA) molecules carry the corresponding amino acids to the ribosome, where they are linked together to form a polypeptide chain. This polypeptide chain then folds into a functional protein.
Types of Genes
Genes are not all created equal. They exhibit significant diversity in their structure, function, and regulation. Some key classifications of genes include:
- Structural Genes: These genes code for proteins that have structural roles within the cell or organism. Examples include genes that code for collagen (a structural protein in connective tissue) or actin (a protein involved in muscle contraction).
- Regulatory Genes: These genes control the expression of other genes. They often code for transcription factors that bind to the promoter regions of other genes, either activating or repressing their transcription.
- Housekeeping Genes: These are genes that are constitutively expressed (always "on") in all cells, providing essential functions for basic cellular processes, such as metabolism and cell division.
- Genes Encoding Non-coding RNAs: Not all genes code for proteins. Some genes code for functional RNA molecules such as ribosomal RNA (rRNA), transfer RNA (tRNA), and microRNAs (miRNAs), which play crucial roles in gene regulation and other cellular processes.
Gene Mutations and Variations
Genes are not static entities; they can undergo changes or mutations. A mutation is any alteration in the DNA sequence of a gene. Mutations can be caused by various factors, including errors during DNA replication, exposure to mutagens (e.g., radiation, certain chemicals), and transposable elements (jumping genes).
Mutations can have a wide range of effects, depending on their location and nature:
- Silent Mutations: These mutations do not alter the amino acid sequence of the protein, having no effect on the protein's function.
- Missense Mutations: These mutations change a single amino acid in the protein, potentially altering its function. The severity of the effect varies greatly, depending on the location and nature of the amino acid change.
- Nonsense Mutations: These mutations introduce a premature stop codon, leading to the production of a truncated, non-functional protein.
- Frameshift Mutations: These mutations involve insertions or deletions of nucleotides that are not multiples of three, shifting the reading frame of the mRNA and causing a completely different amino acid sequence downstream of the mutation. These mutations often result in non-functional proteins.
The Significance of Genes in Heredity
Genes are the fundamental units of inheritance, passed down from parents to offspring through the process of sexual reproduction. Each parent contributes one copy of each gene to their offspring, resulting in two copies of each gene in the offspring (one from each parent). These two copies are called alleles.
Alleles can be identical (homozygous) or different (heterozygous). The combination of alleles an individual possesses for a particular gene determines their genotype, which in turn influences their phenotype. The principles of Mendelian genetics describe the patterns of inheritance of these alleles and the resulting phenotypic ratios.
Mendel's Laws provide a foundational understanding of how genes are inherited:
- Law of Segregation: Each gene has two alleles, one from each parent, and these alleles segregate (separate) during gamete formation (formation of sperm and eggs).
- Law of Independent Assortment: Genes located on different chromosomes are inherited independently of each other.
However, the inheritance patterns of many genes are far more complex than those described by Mendel's laws. Factors such as gene interactions, pleiotropy (one gene affecting multiple traits), and environmental influences can all modify the expression of genes and complicate inheritance patterns.
Beyond Mendelian Genetics: Epigenetics and Gene Regulation
While Mendelian genetics provides a basic framework for understanding inheritance, the field of epigenetics has revealed a layer of complexity that goes beyond the simple sequence of DNA. Epigenetics refers to heritable changes in gene expression that do not involve alterations in the DNA sequence itself. These changes can be influenced by environmental factors, such as diet, stress, and exposure to toxins.
Epigenetic modifications include:
- DNA methylation: The addition of a methyl group to a DNA base, typically cytosine, which often silences gene expression.
- Histone modification: Chemical modifications to histone proteins, around which DNA is wrapped, affecting the accessibility of DNA to transcription machinery.
- Non-coding RNA regulation: The involvement of various non-coding RNA molecules, such as miRNAs, in regulating gene expression.
These epigenetic modifications can be inherited across generations, impacting the phenotype of subsequent generations without altering the underlying DNA sequence.
The Impact of Gene Research on Medicine and Society
The understanding of genes and their functions has revolutionized the fields of medicine and biotechnology. Gene research has led to:
- Genetic Testing and Screening: The ability to identify individuals at risk for certain genetic disorders through genetic testing.
- Gene Therapy: The development of therapies aimed at correcting genetic defects by introducing functional copies of genes into cells.
- Pharmacogenomics: The use of genetic information to personalize drug treatment, optimizing drug efficacy and minimizing side effects.
- Forensic Science: DNA fingerprinting using genetic markers for identifying individuals and solving crimes.
- Agriculture: Genetic engineering to improve crop yields, disease resistance, and nutritional value.
However, the advancements in gene research also raise ethical and societal concerns, such as the potential for genetic discrimination, the misuse of genetic information, and the ethical implications of genetic engineering. Careful consideration of these issues is crucial as gene research continues to progress.
Conclusion: The Ongoing Journey of Genetic Discovery
The gene, as the basic unit of heredity, remains a subject of intense scientific inquiry. Its complexities continue to unfold, revealing new layers of understanding about life's intricate mechanisms. From the intricacies of gene regulation to the far-reaching implications of genetic inheritance, the study of genes is crucial for advancing our knowledge of biology, medicine, and society as a whole. The ongoing journey of genetic discovery holds immense promise for the future, offering potential solutions to some of the most pressing challenges facing humanity, while simultaneously requiring careful ethical consideration to navigate the powerful tools we are developing.
Latest Posts
Latest Posts
-
What Is The Common Name Of The Following Substituent
Apr 24, 2025
-
A Que Temperatura Hierve El Agua En Grados Fahrenheit
Apr 24, 2025
-
What Is The Difference Between Molecular And Ionic Compounds
Apr 24, 2025
-
Why Is Reaching Activation Energy Necessary
Apr 24, 2025
-
What Is The Difference Between A Monosaccharide And Disaccharide
Apr 24, 2025
Related Post
Thank you for visiting our website which covers about The Basic Unit Of Heredity Is The . We hope the information provided has been useful to you. Feel free to contact us if you have any questions or need further assistance. See you next time and don't miss to bookmark.