The Coiled Structure Made Of Dna And Proteins Are
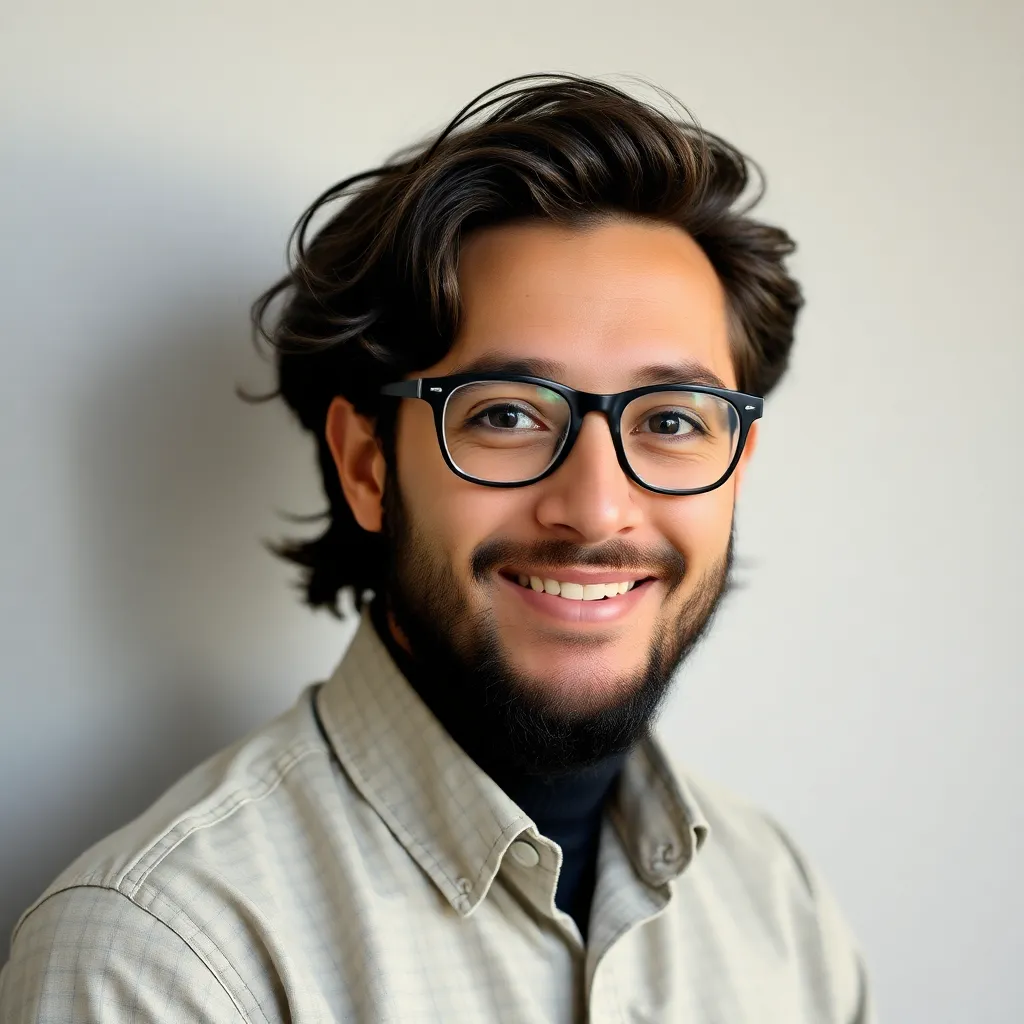
Muz Play
Mar 12, 2025 · 7 min read

Table of Contents
The Coiled Structure Made of DNA and Proteins: A Deep Dive into Chromatin
The fundamental unit of heredity, deoxyribonucleic acid (DNA), doesn't exist in a naked, free-floating state within the cell nucleus. Instead, this incredibly long molecule is meticulously packaged and organized into a compact, highly structured form known as chromatin. Understanding the intricate architecture of chromatin is crucial to comprehending not only how DNA is stored but also how it's accessed, replicated, repaired, and regulated during the cell cycle. This article will delve into the fascinating world of chromatin, exploring its various levels of organization, the proteins involved, and the functional implications of its structure.
The Building Blocks: DNA and Histones
At the most basic level, chromatin is composed of DNA and a family of proteins called histones. DNA, the blueprint of life, is a double-stranded helix carrying the genetic code. Histones, on the other hand, are small, positively charged proteins that are crucial for DNA packaging. The positive charge of histones allows them to interact strongly with the negatively charged phosphate backbone of DNA.
Histone Octamer: The Nucleosome Core
The fundamental repeating unit of chromatin is the nucleosome. A nucleosome consists of approximately 147 base pairs of DNA wrapped around a histone octamer. This octamer is composed of two copies each of four core histone proteins: H2A, H2B, H3, and H4. Imagine the DNA wrapping around the histone octamer like thread around a spool. This wrapping compacts the DNA significantly, reducing its length and making it more manageable within the nucleus.
Linker DNA and Histone H1
Between each nucleosome core is a stretch of DNA called linker DNA. The length of linker DNA varies depending on the organism and the cell type. Another histone protein, H1, plays a key role in stabilizing the nucleosome structure and compacting the linker DNA. H1 sits on the outside of the nucleosome core, binding to both the linker DNA and the DNA where it enters and exits the core particle. It acts as a clamp, further condensing the chromatin fiber.
Higher-Order Chromatin Structure: From Nucleosomes to Chromosomes
The nucleosome represents the first level of DNA compaction, but chromatin organization extends far beyond this basic unit. The nucleosomes themselves are arranged into higher-order structures, further compacting the DNA to fit within the confines of the cell nucleus.
The 30-nm Fiber: A Solenoid or Zigzag?
The next level of organization involves the arrangement of nucleosomes into a more compact fiber approximately 30 nanometers in diameter. The exact structure of this 30-nm fiber remains a subject of debate, with two main models proposed: the solenoid model and the zigzag model. In the solenoid model, nucleosomes are arranged in a helical structure, resembling a solenoid. In the zigzag model, nucleosomes are arranged in a more irregular, zigzag pattern. Regardless of the precise structure, this level of organization further condenses the chromatin.
Chromatin Loops and Topologically Associating Domains (TADs)
Beyond the 30-nm fiber, chromatin is organized into loops and domains. These loops are formed by interactions between different regions of the chromatin fiber, often mediated by proteins that bind to specific DNA sequences. Topologically associating domains (TADs) are larger, megabase-sized regions of the genome that exhibit high levels of internal interaction but limited interaction with neighboring TADs. These TADs are thought to play a crucial role in regulating gene expression by keeping enhancers and promoters within the same functional unit.
Chromosomes: The Ultimate Level of Condensation
The ultimate level of chromatin organization is the chromosome. During cell division, chromatin undergoes extreme condensation, forming the highly compact structures we recognize as chromosomes. This condensation is essential for accurate segregation of the genetic material during mitosis and meiosis. The process involves further compaction of the chromatin fiber, with the assistance of additional structural proteins, ultimately resulting in the highly condensed chromosome structure visible under a light microscope.
Chromatin Remodeling and Gene Regulation
The structure of chromatin isn't static; it's dynamically regulated in response to various cellular signals. This dynamic regulation is crucial for controlling gene expression. Chromatin remodeling complexes, composed of multiple proteins, can alter the structure of chromatin by repositioning or evicting nucleosomes. This process can expose or hide DNA sequences, influencing the accessibility of the transcriptional machinery and thereby regulating gene expression.
Histone Modifications: The Histone Code
Histone proteins can be modified post-translationally, meaning that chemical groups are added or removed from the amino acid side chains of the histone tails. These modifications, collectively known as the histone code, include acetylation, methylation, phosphorylation, and ubiquitination. These modifications can alter the interaction between histones and DNA, influencing chromatin structure and accessibility. For example, histone acetylation generally leads to a more open chromatin conformation, promoting gene expression, while histone methylation can have either activating or repressive effects, depending on the specific amino acid residue and the number of methyl groups added.
Chromatin-Associated Proteins: Beyond Histones
In addition to histones, many other proteins associate with chromatin, playing crucial roles in regulating gene expression and maintaining chromatin structure. These proteins include transcription factors, which bind to specific DNA sequences and regulate gene transcription, and chromatin remodeling complexes, which alter the structure of chromatin. Other proteins are involved in DNA replication, repair, and recombination.
Chromatin and Disease
Dysregulation of chromatin structure and function is implicated in a wide range of human diseases, including cancer, neurological disorders, and immune deficiencies. Aberrant chromatin remodeling, histone modifications, and altered expression of chromatin-associated proteins can disrupt gene expression, leading to cellular dysfunction and disease. Cancer, in particular, is often characterized by widespread alterations in chromatin structure, promoting uncontrolled cell growth and proliferation.
Cancer and Chromatin Dysregulation
Numerous studies have linked cancer development to alterations in chromatin structure and function. For example, mutations in genes encoding histone-modifying enzymes or chromatin remodeling complexes are frequently observed in various cancer types. These mutations can lead to aberrant gene expression, contributing to tumorigenesis. Moreover, changes in the 3D organization of the genome, such as disruptions in TADs, have been associated with altered gene expression patterns in cancer cells. Understanding the role of chromatin in cancer pathogenesis is crucial for developing novel therapeutic strategies.
Neurological Disorders and Chromatin
Neurological disorders are also often associated with defects in chromatin regulation. For example, mutations in genes encoding histone-modifying enzymes have been linked to intellectual disability and neurodevelopmental disorders. Changes in chromatin structure can affect the expression of genes essential for neuronal development and function, leading to neurological deficits. Research continues to uncover the complex interplay between chromatin and neurological diseases, providing potential avenues for developing therapeutic interventions.
Future Directions in Chromatin Research
The study of chromatin is a rapidly evolving field. Advancements in technologies, such as high-throughput sequencing and advanced microscopy techniques, are providing unprecedented insights into the intricate architecture and dynamic regulation of chromatin. Future research will likely focus on:
- High-resolution mapping of chromatin structure: Determining the precise three-dimensional organization of chromatin at increasingly higher resolutions.
- Understanding the interplay between chromatin structure and gene regulation: Investigating the mechanisms by which chromatin structure influences gene expression and how this regulation is affected by various cellular signals and environmental factors.
- Developing novel therapeutic strategies targeting chromatin: Designing drugs that can specifically target aberrant chromatin structures or processes implicated in disease.
- Exploring the role of chromatin in aging and age-related diseases: Investigating how chromatin changes with age and its contribution to age-related diseases.
In conclusion, the coiled structure made of DNA and proteins, chromatin, is far more than just a compact packaging system for DNA. It's a highly dynamic and intricately regulated structure that plays a crucial role in every aspect of genome function, from gene expression to DNA replication and repair. Further research into the complexities of chromatin will undoubtedly provide essential insights into the fundamental processes of life and lead to the development of new therapies for a wide range of diseases. The field is ripe with discoveries waiting to be made, promising a deeper understanding of this fundamental component of life.
Latest Posts
Latest Posts
-
How To Write An Ionization Equation
May 09, 2025
-
How To Go From Alkene To Alkyne
May 09, 2025
-
Trial Balance Balance Sheet And Income Statement
May 09, 2025
-
Reproductive Isolation Reproductive Isolation Is Classified As Whether It
May 09, 2025
-
Definition Of Subtraction Property Of Equality
May 09, 2025
Related Post
Thank you for visiting our website which covers about The Coiled Structure Made Of Dna And Proteins Are . We hope the information provided has been useful to you. Feel free to contact us if you have any questions or need further assistance. See you next time and don't miss to bookmark.