The Movement Of Materials From Low To High Concentration
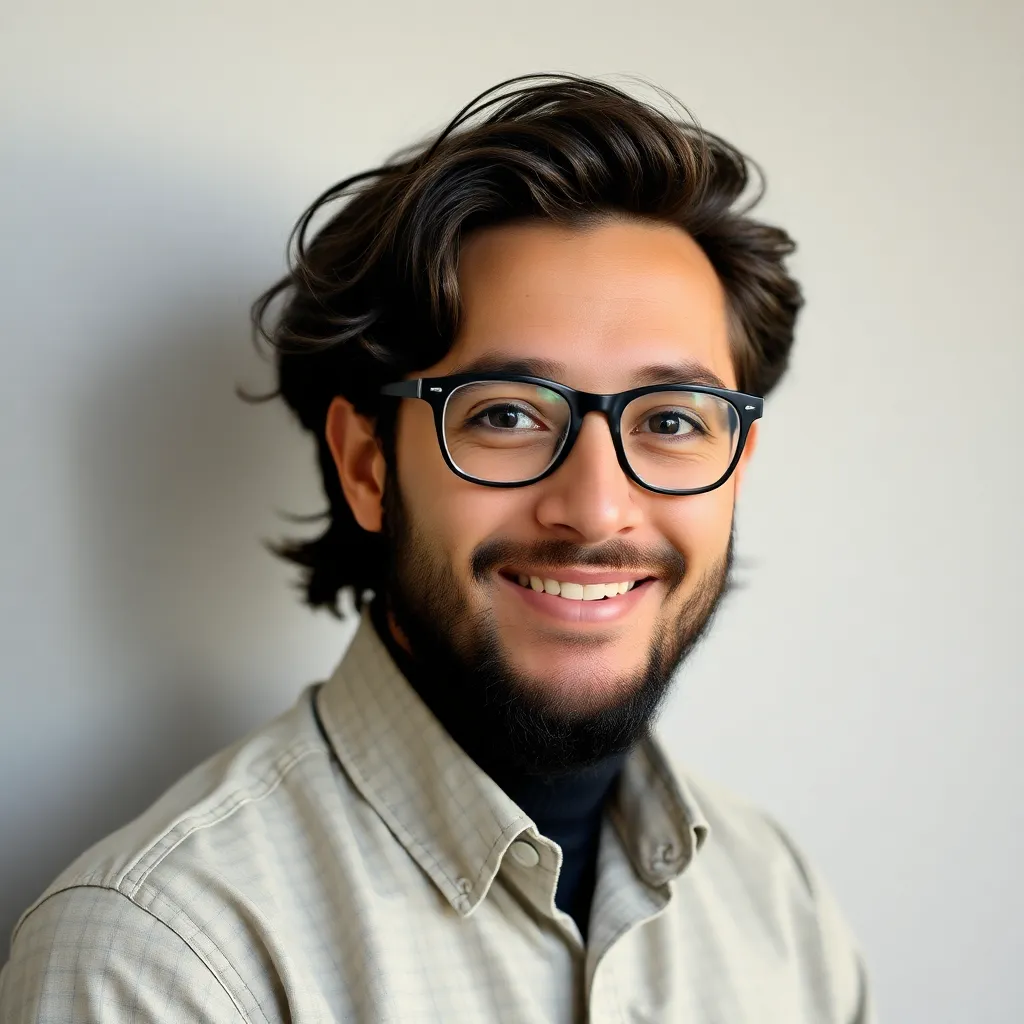
Muz Play
Apr 11, 2025 · 6 min read

Table of Contents
The Movement of Materials from Low to High Concentration: Understanding Active Transport
The movement of materials across cell membranes is fundamental to life. While passive transport mechanisms like diffusion and osmosis move substances down their concentration gradients (from high to low concentration), requiring no energy input, the movement of materials from a region of low concentration to a region of high concentration requires energy. This process, known as active transport, is crucial for maintaining cellular homeostasis and carrying out various vital functions. This article will delve deep into the mechanisms, significance, and various examples of active transport, exploring its intricate role in biological systems.
Understanding Concentration Gradients
Before delving into the complexities of active transport, it's essential to understand the concept of a concentration gradient. A concentration gradient is the difference in the concentration of a substance between two areas. Substances naturally tend to move down their concentration gradient, from an area of high concentration to an area of low concentration, a process driven by entropy—the tendency towards increased disorder. This passive movement continues until equilibrium is reached, where the concentration is uniform throughout. Active transport, however, works against this natural tendency, requiring energy expenditure to move substances against their concentration gradient.
The Mechanics of Active Transport: Pumping Against the Tide
Active transport mechanisms employ specialized transmembrane proteins, often called pumps, to move molecules or ions against their concentration gradient. These pumps utilize energy, primarily in the form of adenosine triphosphate (ATP), to drive the transport process. The energy from ATP hydrolysis fuels conformational changes in the pump protein, enabling it to bind to the transported substance on one side of the membrane, undergo a shape change, and release the substance on the other side.
There are two main types of active transport:
1. Primary Active Transport: Direct ATP Hydrolysis
Primary active transport directly uses ATP hydrolysis to power the movement of substances. A classic example is the sodium-potassium pump (Na+/K+ ATPase), a ubiquitous protein found in animal cell membranes. This pump maintains the characteristic electrochemical gradient across the cell membrane, crucial for nerve impulse transmission, muscle contraction, and nutrient uptake. For every molecule of ATP hydrolyzed, the pump moves three sodium ions (Na+) out of the cell and two potassium ions (K+) into the cell, both against their concentration gradients. This creates a higher concentration of Na+ outside the cell and a higher concentration of K+ inside the cell.
Another example of primary active transport involves the proton pump (H+ ATPase), which is vital for maintaining pH gradients across membranes in various organisms. This pump actively transports protons (H+) across the membrane, creating a proton motive force that can be used to drive other processes, such as ATP synthesis during cellular respiration.
2. Secondary Active Transport: Piggybacking on Existing Gradients
Secondary active transport indirectly utilizes ATP. It leverages the energy stored in pre-existing electrochemical gradients created by primary active transport. This type of transport often involves the co-transport of two substances: one moving down its concentration gradient (providing the energy) and the other moving against its concentration gradient.
There are two subtypes of secondary active transport:
-
Symport: Both substances move in the same direction across the membrane. For example, the sodium-glucose cotransporter (SGLT) in the intestines uses the energy from the downhill movement of Na+ (from high to low concentration outside the cell) to transport glucose against its concentration gradient into the cell.
-
Antiport: Substances move in opposite directions across the membrane. The sodium-calcium exchanger (NCX) in heart muscle cells is an excellent example. It uses the inward movement of Na+ to pump Ca2+ out of the cell, maintaining the low intracellular Ca2+ concentration necessary for proper muscle function.
The Significance of Active Transport in Biological Systems
Active transport plays a critical role in numerous cellular processes, including:
-
Maintaining Cell Volume: Active transport regulates the movement of water and solutes, preventing osmotic lysis or shrinkage.
-
Nutrient Uptake: Cells actively transport essential nutrients like glucose, amino acids, and minerals against their concentration gradients to maintain adequate intracellular levels.
-
Waste Removal: Active transport systems remove metabolic waste products from cells, preventing their accumulation and maintaining cellular homeostasis.
-
Signal Transduction: Active transport is involved in the movement of ions across membranes, crucial for generating electrical signals in nerve and muscle cells.
-
Maintaining Ion Gradients: As seen with the Na+/K+ pump, active transport maintains electrochemical gradients that are essential for various cellular processes.
-
Endocytosis and Exocytosis: These processes, while not strictly active transport, often rely on active transport mechanisms to move substances into or out of vesicles. For instance, the uptake of macromolecules via receptor-mediated endocytosis requires energy-dependent processes.
-
Pharmacology: Many drugs rely on active transport systems for their uptake and distribution within the body, influencing their efficacy and pharmacokinetic properties.
Examples of Active Transport in Different Organisms
Active transport is not limited to animal cells; it is a ubiquitous process found throughout the biological world:
-
Plants: Plants utilize active transport to absorb minerals from the soil, against their concentration gradients, and to transport sugars from leaves to other parts of the plant. Proton pumps are particularly important in plant cells for driving nutrient uptake.
-
Bacteria: Bacterial cells employ active transport mechanisms for nutrient acquisition and maintaining osmotic balance. Many bacteria use proton pumps to create a proton motive force, used for various purposes, including ATP synthesis and nutrient uptake.
-
Fungi: Fungi also utilize active transport for the absorption of nutrients from their environment, often facing nutrient-poor conditions.
-
Archaea: These extremophiles often possess unique active transport systems adapted to their harsh environments, enabling them to survive in extreme temperatures, salinity, or pH levels.
Active Transport and Disease
Dysfunction in active transport systems can lead to various diseases. For instance, mutations in genes encoding the Na+/K+ pump can cause several cardiac and neurological disorders. Problems with glucose transport across cell membranes can contribute to diabetes. Furthermore, some bacterial infections involve the disruption of host cell active transport systems.
Technological Applications: Mimicking Nature's Efficiency
Scientists are actively exploring the principles of active transport to develop novel technologies. Researchers are developing artificial membrane systems that mimic the function of biological pumps, potentially leading to new drug delivery systems, biosensors, and water purification technologies. The ability to precisely control the movement of substances against their concentration gradients offers exciting possibilities for various applications.
Conclusion: The Indispensable Role of Active Transport
Active transport is a fundamental process that underpins virtually all aspects of cellular life. Its ability to move substances against their concentration gradients enables cells to maintain homeostasis, acquire essential nutrients, expel waste, and generate electrical signals. The intricate mechanisms of active transport, ranging from primary to secondary active transport, demonstrate the remarkable efficiency and complexity of biological systems. Understanding active transport is crucial not only for comprehending basic biological principles but also for developing new technologies and treatments for various diseases. Further research into this fascinating area will undoubtedly continue to unveil its multifaceted role in life's processes.
Latest Posts
Latest Posts
-
Disk Washer And Shell Method Formulas
Apr 18, 2025
-
Arrange The Molecule In The Order Of Increasing Boiling Point
Apr 18, 2025
-
The Chemical Reaction Of 2 Butene And Hcl Yields What Product
Apr 18, 2025
-
Which Formula Represents An Ionic Compound
Apr 18, 2025
-
The Chemical Formula For An Ionic Compound Represents The
Apr 18, 2025
Related Post
Thank you for visiting our website which covers about The Movement Of Materials From Low To High Concentration . We hope the information provided has been useful to you. Feel free to contact us if you have any questions or need further assistance. See you next time and don't miss to bookmark.