The Phylogenetic Classification Of Bacteria Is Based On
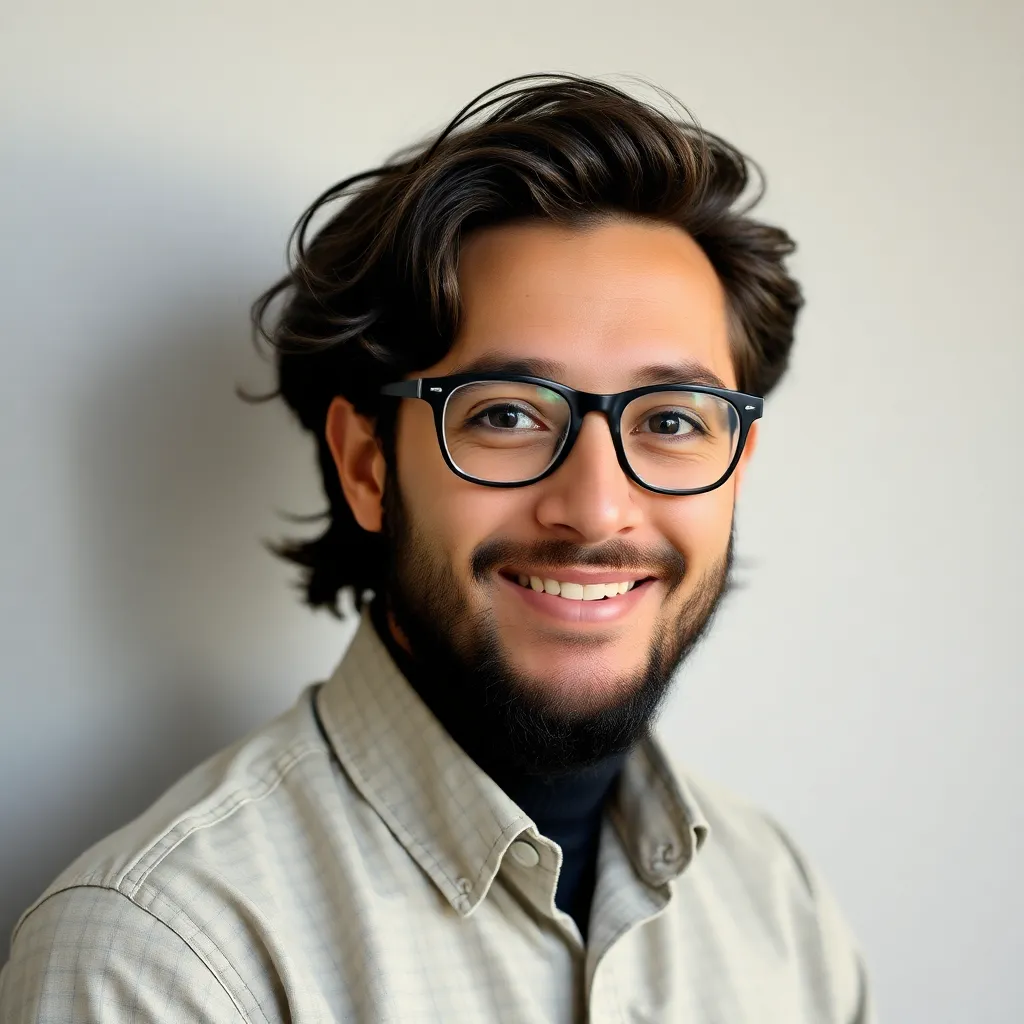
Muz Play
Apr 03, 2025 · 6 min read

Table of Contents
The Phylogenetic Classification of Bacteria is Based On: A Deep Dive into 16S rRNA and Beyond
The classification of bacteria, those microscopic workhorses of the biological world, has undergone a dramatic revolution thanks to advancements in molecular biology. Gone are the days of relying solely on observable phenotypic characteristics, like morphology or metabolic capabilities, which often proved insufficient to accurately reflect evolutionary relationships. Today, the phylogenetic classification of bacteria is primarily based on genomic data, with the 16S ribosomal RNA (rRNA) gene serving as the gold standard. However, the field continues to evolve, incorporating more sophisticated techniques and broader genomic information to create a more comprehensive and accurate bacterial tree of life.
The Reign of 16S rRNA: A Molecular Clock for Bacteria
The 16S rRNA gene holds a unique position in bacterial classification due to several crucial properties:
- Universality: This gene is present in virtually all bacteria, making it a suitable marker for comparative analysis across diverse taxa.
- Conservation: Certain regions of the 16S rRNA gene are highly conserved, indicating common ancestry and allowing for the alignment of sequences from distantly related bacteria.
- Variation: Other regions exhibit sufficient variability to distinguish between different bacterial species and even strains. This variation reflects the evolutionary divergence of different bacterial lineages.
This combination of conserved and variable regions makes 16S rRNA an ideal molecular clock. By comparing the sequences of this gene in different bacteria, scientists can infer the evolutionary distances between them and construct phylogenetic trees that illustrate their evolutionary relationships. The more similar the 16S rRNA sequences, the more closely related the bacteria are presumed to be.
The 16S rRNA Sequencing Process and its Applications:
The process generally involves:
- DNA Extraction: Isolating bacterial DNA from a sample.
- PCR Amplification: Specifically amplifying the 16S rRNA gene using primers designed to target conserved regions.
- Sequencing: Determining the precise order of nucleotides in the amplified 16S rRNA gene.
- Sequence Alignment and Phylogenetic Analysis: Comparing the obtained sequence with databases of known 16S rRNA sequences to identify the closest relatives and construct phylogenetic trees using various algorithms (e.g., neighbor-joining, maximum likelihood, Bayesian inference).
The applications of 16S rRNA sequencing are vast and impact many fields:
- Microbial Ecology: Analyzing the composition of microbial communities in various environments (soil, water, human gut) through high-throughput sequencing (metagenomics).
- Clinical Microbiology: Identifying bacterial pathogens in clinical samples for accurate diagnosis and treatment.
- Taxonomy and Systematics: Establishing the evolutionary relationships between bacteria and refining the taxonomic classification of bacterial species.
- Food Microbiology: Monitoring the microbial quality and safety of food products.
Beyond 16S rRNA: A Multi-Gene Approach to Phylogenetic Resolution
While 16S rRNA has been instrumental in shaping our understanding of bacterial phylogeny, it has limitations. The relatively slow rate of evolution in this gene can lead to insufficient resolution for closely related species or strains. Furthermore, horizontal gene transfer (HGT) – the movement of genetic material between organisms – can confound phylogenetic analysis based on a single gene. To address these limitations, a multi-gene approach is increasingly employed.
This approach involves analyzing the sequences of multiple genes simultaneously, ideally selecting genes with different evolutionary rates. By combining data from several genes, scientists can obtain a more robust and accurate phylogenetic reconstruction that mitigates the effects of HGT and improves resolution at finer taxonomic levels.
Some commonly used genes in multi-gene phylogenetic analyses include:
- 23S rRNA: Another ribosomal RNA gene with a slower evolutionary rate than 16S rRNA.
- rpoB: The gene encoding the beta subunit of RNA polymerase.
- gyrB: The gene encoding the gyrase B subunit, involved in DNA supercoiling.
- atpD: The gene encoding the ATP synthase subunit.
The combination of data from these and other genes provides a more holistic view of bacterial evolution. This approach is particularly crucial for resolving the phylogenetic relationships among closely related bacteria where 16S rRNA alone may provide insufficient discriminatory power.
Genomic Approaches: Unveiling the Bacterial Genome's Secrets
The advent of affordable whole-genome sequencing has revolutionized bacterial phylogenetics. Analyzing entire genomes allows for a far more comprehensive assessment of evolutionary relationships compared to using a limited number of genes. Whole-genome phylogenetic analyses leverage various genomic features, including:
- Gene Content: The presence or absence of specific genes can indicate evolutionary relationships, especially in the context of adaptation to different environmental niches.
- Genome Size and GC Content: These characteristics can reflect the evolutionary history of bacterial lineages.
- Synteny: The order of genes on the chromosome can be highly conserved in closely related species, providing another piece of evolutionary information.
- Horizontally Transferred Genes: While HGT can complicate phylogenetic analysis, careful identification and removal of horizontally transferred genes can provide a clearer picture of the core bacterial genome and evolutionary relationships.
Advanced Phylogenetic Methods: Improving Accuracy and Resolution
The analysis of large genomic datasets necessitates sophisticated computational methods. These include:
- Supertree Methods: These methods combine phylogenetic information from different genes or datasets to construct a comprehensive phylogeny.
- Bayesian Phylogenetic Inference: This statistical approach considers uncertainty in phylogenetic relationships and provides probability estimates for different evolutionary scenarios.
- Maximum Likelihood Methods: These methods search for the phylogenetic tree that is most likely to have generated the observed data.
The Challenges and Future Directions of Bacterial Phylogenetics
Despite significant progress, challenges remain in bacterial phylogenetic classification:
- The vast diversity of bacteria: The sheer number of bacterial species, many of which remain uncultured, poses a significant challenge to complete phylogenetic analysis.
- Horizontal gene transfer: HGT continues to confound phylogenetic analyses, requiring sophisticated methods to disentangle its impact.
- Rapid evolution of certain bacterial lineages: The rapid evolution of certain bacterial lineages can lead to difficulties in resolving their phylogenetic relationships.
- Data interpretation and curation: The sheer volume of data generated by whole-genome sequencing requires robust computational and statistical methods for analysis and interpretation, and accurate data curation is crucial.
Future directions in bacterial phylogenetics include:
- Metagenomic approaches: Analyzing the complete genomic content of microbial communities directly from environmental samples without the need for culturing.
- Single-cell genomics: Sequencing the genomes of individual bacterial cells, even from uncultivable species.
- Improved phylogenetic algorithms: Developing more powerful and sophisticated computational methods to handle large genomic datasets and account for the complexities of bacterial evolution.
- Integration of multiple data types: Combining genomic data with other types of data, such as phenotypic characteristics and ecological information, to obtain a more complete picture of bacterial evolution.
Conclusion: A Dynamic and Ever-Evolving Field
The phylogenetic classification of bacteria has evolved considerably, moving from reliance on observable traits to the use of sophisticated molecular and genomic techniques. While 16S rRNA remains a cornerstone, the integration of multi-gene approaches and whole-genome sequencing provides much greater resolution and accuracy. The field continues to be dynamic, with ongoing development of new methodologies and the constant exploration of bacterial diversity, promising a far more comprehensive and accurate understanding of the bacterial tree of life in the years to come. The ongoing advancements in technology and computational biology will undoubtedly continue to refine our understanding of bacterial evolution and reshape our classification systems. This continuous evolution of the field underscores the complexity and fascinating nature of the microbial world.
Latest Posts
Latest Posts
-
What Are The Basic Building Blocks Of Nucleic Acids
Apr 04, 2025
-
Does Wedge Mean Axial Or Equatorial
Apr 04, 2025
-
Label The Indicated Body Surface Regions
Apr 04, 2025
-
Is Hydrogen A Nonmetal Metal Or Metalloid
Apr 04, 2025
-
Gas At Room Temperature Physical Or Chemical
Apr 04, 2025
Related Post
Thank you for visiting our website which covers about The Phylogenetic Classification Of Bacteria Is Based On . We hope the information provided has been useful to you. Feel free to contact us if you have any questions or need further assistance. See you next time and don't miss to bookmark.