The Quantitative Proportion Of Reactants To Products
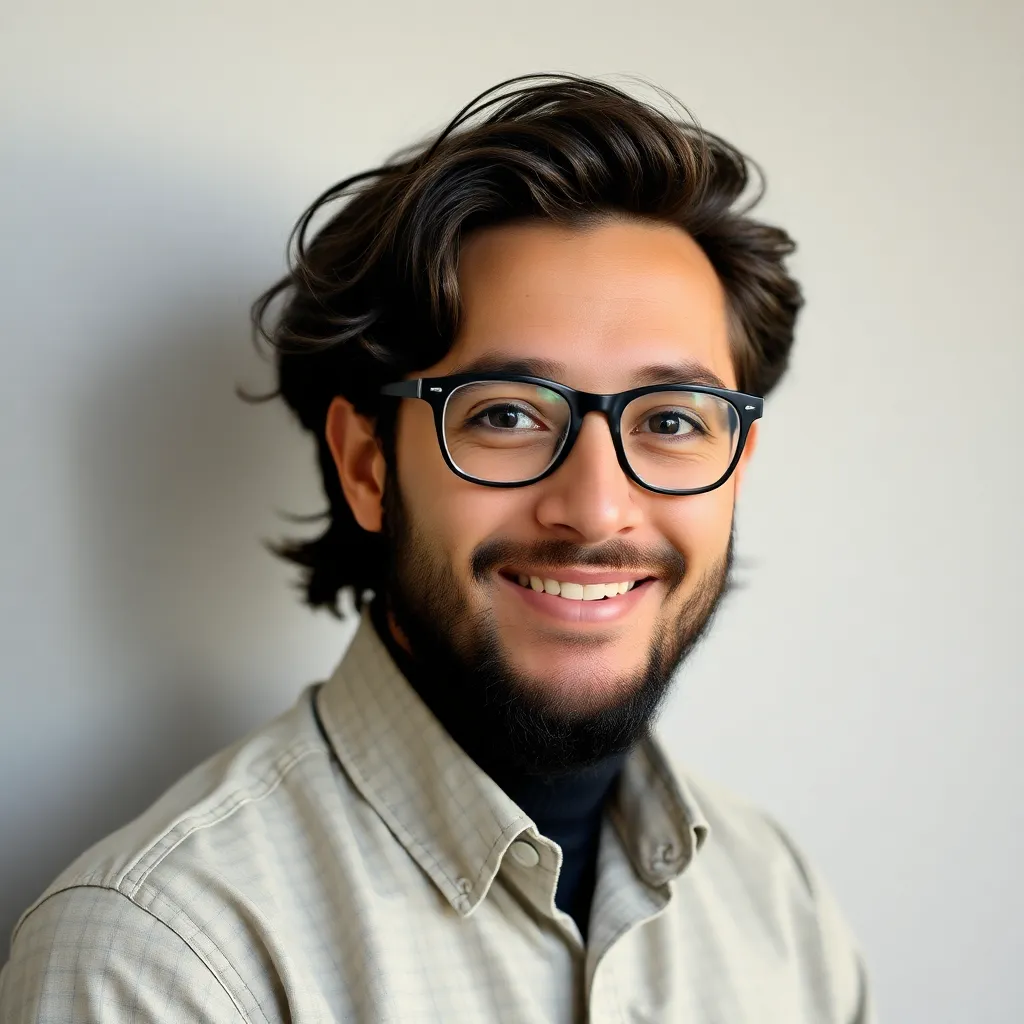
Muz Play
Apr 26, 2025 · 6 min read

Table of Contents
The Quantitative Proportion of Reactants to Products: Stoichiometry and Beyond
Stoichiometry, at its core, is the study of the quantitative relationships between reactants and products in a chemical reaction. Understanding these relationships is fundamental to chemistry, allowing us to predict the amount of product formed from a given amount of reactants, determine the limiting reactant, and calculate the theoretical and percent yields of a reaction. This article delves deep into the quantitative proportion of reactants to products, exploring the concepts of stoichiometry, limiting reactants, percent yield, and the real-world applications of these principles.
Understanding the Basics of Stoichiometry
Stoichiometry relies heavily on the law of conservation of mass, which states that matter cannot be created or destroyed in a chemical reaction. This means that the total mass of reactants must equal the total mass of products. This fundamental principle forms the bedrock of all stoichiometric calculations.
To perform stoichiometric calculations effectively, we need a balanced chemical equation. This equation represents the reaction using chemical formulas, showing the relative amounts of reactants and products involved. The coefficients in the balanced equation provide the mole ratio between the substances, which is crucial for quantitative analysis.
For example, consider the combustion of methane:
CH₄ + 2O₂ → CO₂ + 2H₂O
This equation tells us that one mole of methane (CH₄) reacts with two moles of oxygen (O₂) to produce one mole of carbon dioxide (CO₂) and two moles of water (H₂O). These coefficients are the key to determining the quantitative relationships.
Mole Ratios and Calculations
The mole ratio is the ratio of the coefficients in a balanced chemical equation. This ratio allows us to convert between moles of one substance and moles of another substance in the reaction. For instance, in the methane combustion example:
- The mole ratio of CH₄ to CO₂ is 1:1.
- The mole ratio of O₂ to H₂O is 2:2 (or 1:1).
- The mole ratio of CH₄ to O₂ is 1:2.
These ratios are essential for performing stoichiometric calculations, allowing us to determine the amount of product formed from a known amount of reactant or vice-versa.
Determining the Limiting Reactant
In most real-world scenarios, reactants are not present in the exact stoichiometric ratios indicated by the balanced equation. One reactant will be completely consumed before others, limiting the amount of product formed. This reactant is called the limiting reactant. Identifying the limiting reactant is crucial for optimizing reaction yields.
To determine the limiting reactant, we can use the mole ratios from the balanced equation. We calculate the amount of product that would be formed if each reactant were completely consumed. The reactant that produces the least amount of product is the limiting reactant.
Example: Limiting Reactant Calculation
Let's say we have 10 grams of methane (CH₄) and 50 grams of oxygen (O₂) reacting according to the balanced equation above. To find the limiting reactant:
-
Convert grams to moles: Using the molar masses of CH₄ (16 g/mol) and O₂ (32 g/mol), we find we have 0.625 moles of CH₄ and 1.56 moles of O₂.
-
Use mole ratios: According to the balanced equation, 1 mole of CH₄ reacts with 2 moles of O₂. Therefore, 0.625 moles of CH₄ would require 1.25 moles of O₂ (0.625 mol CH₄ × 2 mol O₂/1 mol CH₄). Since we have 1.56 moles of O₂, we have enough oxygen.
-
Determine the limiting reactant: If we use all the methane, we can produce 0.625 moles of CO₂. If we use all the oxygen, we can produce 0.78 moles of CO₂ (1.56 mol O₂ × 1 mol CO₂/2 mol O₂). Since using all the methane produces less CO₂, methane is the limiting reactant.
Calculating Percent Yield
The theoretical yield is the maximum amount of product that can be formed based on the stoichiometry of the reaction and the amount of limiting reactant. However, in reality, the actual amount of product obtained (the actual yield) is often less than the theoretical yield due to various factors, such as incomplete reactions, side reactions, or loss of product during purification.
The percent yield is a measure of the efficiency of the reaction and is calculated as:
Percent Yield = (Actual Yield / Theoretical Yield) × 100%
A high percent yield indicates a more efficient reaction. A low percent yield suggests potential problems with the reaction conditions or procedure.
Advanced Stoichiometric Concepts
Beyond the basic principles, several advanced concepts enhance our understanding of quantitative relationships in chemical reactions:
Stoichiometry of Solutions
When reactants are dissolved in solutions, the concentration of the solutions (usually expressed in molarity) is essential for stoichiometric calculations. Molarity is defined as moles of solute per liter of solution. We use molarity to calculate the number of moles of reactant present in a given volume of solution.
Gas Stoichiometry
Gases behave differently than solids and liquids. Their volumes are significantly affected by temperature and pressure. The ideal gas law (PV = nRT) is crucial for gas stoichiometry calculations. We can use this law to determine the volume of a gas involved in a reaction, given its pressure, temperature, and number of moles.
Thermochemistry and Stoichiometry
Thermochemistry combines stoichiometry with thermodynamics. We can use stoichiometry to calculate the heat released or absorbed during a reaction, using the enthalpy change (ΔH) of the reaction.
Real-World Applications of Stoichiometry
Stoichiometry is not just a theoretical concept; it has numerous practical applications in various fields:
-
Industrial Chemistry: Stoichiometry is vital for optimizing chemical processes in industries such as fertilizers, pharmaceuticals, and plastics. It helps determine the optimal amounts of reactants to maximize product yield and minimize waste.
-
Environmental Science: Stoichiometry is used to assess the impact of pollutants on the environment and develop strategies for remediation. For example, it helps determine the amount of oxygen required to decompose organic waste.
-
Analytical Chemistry: Stoichiometry is crucial for quantitative analysis, allowing chemists to determine the composition of samples using titration and other analytical techniques.
-
Biochemistry: Stoichiometry is essential for understanding metabolic pathways and calculating the amounts of enzymes and substrates required for biochemical reactions.
Conclusion
Stoichiometry is a cornerstone of chemistry, providing a quantitative framework for understanding chemical reactions. From determining limiting reactants and calculating percent yield to understanding advanced concepts like gas stoichiometry and thermochemistry, the principles of stoichiometry have wide-ranging applications in various scientific and industrial fields. Mastering stoichiometry is crucial for anyone seeking a deeper understanding of chemical processes and their practical implications. The ability to accurately predict and control the quantitative relationships between reactants and products is essential for success in countless scientific endeavors and industrial processes. Further exploration into the intricacies of stoichiometry will undoubtedly reveal its profound influence on numerous aspects of our lives, from the products we use daily to the environmental sustainability of our planet.
Latest Posts
Latest Posts
-
The Size Of A Eukaryotic Cell Ribosome Is
Apr 26, 2025
-
How Is Drama Different From Other Kinds Of Fiction
Apr 26, 2025
-
11 3 Practice Dividing Polynomials Form G Answers
Apr 26, 2025
-
How Many Sides A Quadrilateral Have
Apr 26, 2025
-
The Olfactory Bulbs Of The Sheep
Apr 26, 2025
Related Post
Thank you for visiting our website which covers about The Quantitative Proportion Of Reactants To Products . We hope the information provided has been useful to you. Feel free to contact us if you have any questions or need further assistance. See you next time and don't miss to bookmark.