The Strands In A Double Helix Of Dna Are
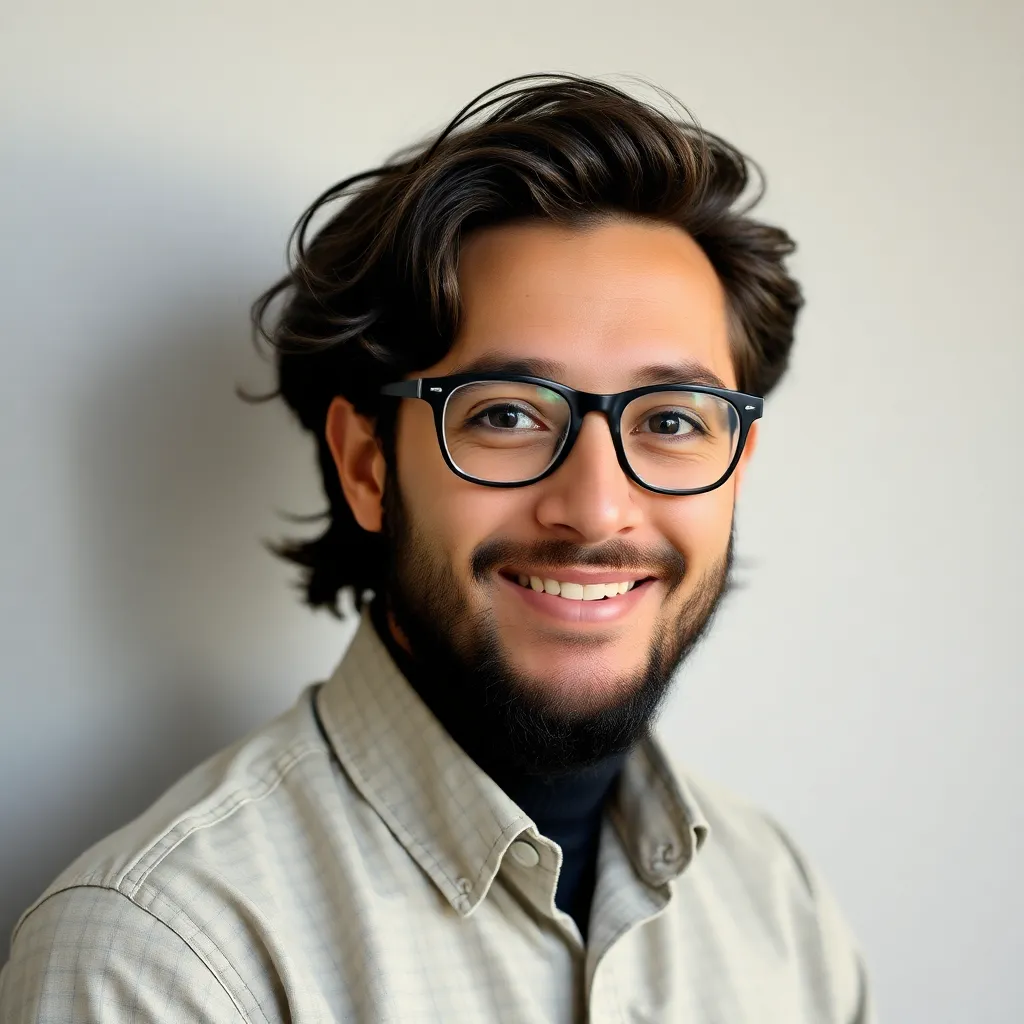
Muz Play
May 10, 2025 · 6 min read
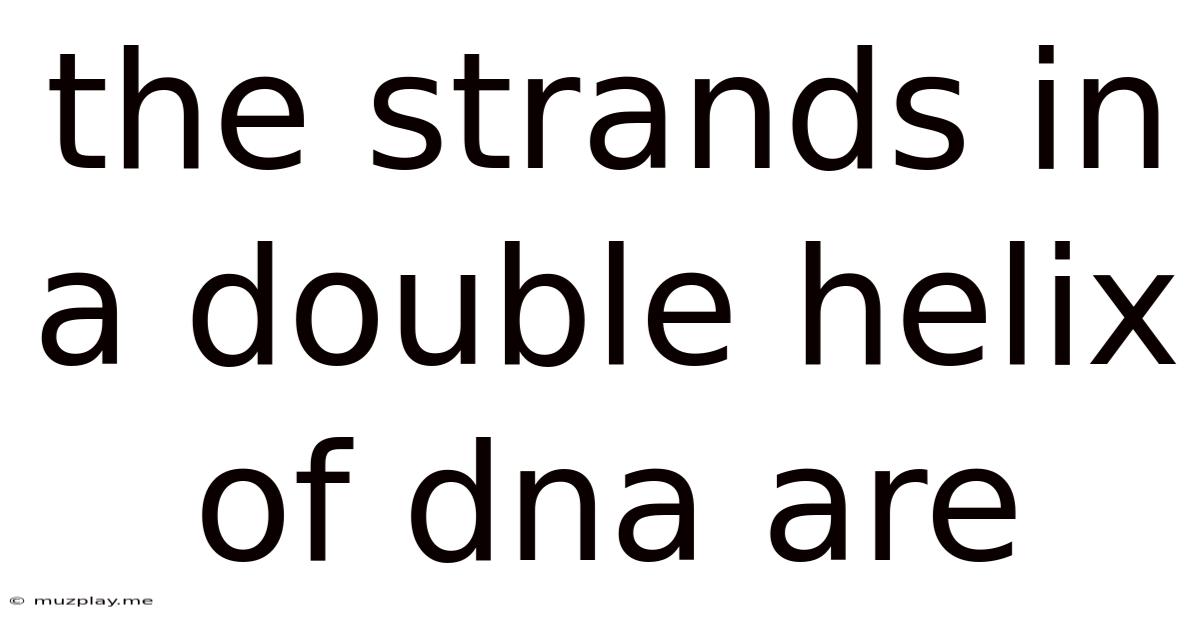
Table of Contents
The Strands in a Double Helix of DNA: A Deep Dive into Structure and Function
Deoxyribonucleic acid, or DNA, is the fundamental blueprint of life. Its iconic double helix structure, discovered by Watson and Crick in 1953, revolutionized our understanding of heredity and opened doors to countless advancements in biology and medicine. But beyond the elegant visual representation, lies a complex interplay of molecular components and forces that dictate DNA's incredible functionality. This article delves into the intricate details of the strands within the DNA double helix, exploring their composition, interactions, and the crucial roles they play in cellular processes.
The Building Blocks: Nucleotides and their Arrangement
The DNA double helix is not a simple, uniform structure. Its very essence lies in the specific arrangement of its fundamental building blocks: nucleotides. Each nucleotide consists of three components:
- A deoxyribose sugar: A five-carbon sugar that forms the backbone of the strand.
- A phosphate group: This negatively charged group links the sugar molecules together, creating the sugar-phosphate backbone. The negative charge plays a significant role in DNA's stability and interactions with proteins.
- A nitrogenous base: This is where the crucial genetic information resides. There are four nitrogenous bases in DNA: adenine (A), guanine (G), cytosine (C), and thymine (T). These bases are categorized as either purines (A and G, with double-ring structures) or pyrimidines (C and T, with single-ring structures).
The sequence of these nitrogenous bases along the DNA strand determines the genetic code. The order of A, T, G, and C dictates the instructions for building proteins and regulating gene expression. This sequence is what differentiates one organism from another and even one individual from another.
Antiparallel Strands: A Key to the Double Helix's Stability
The two strands of the DNA double helix are not parallel; they run antiparallel to each other. This means that one strand runs in the 5' to 3' direction, while the other runs in the 3' to 5' direction. The 5' and 3' designations refer to the carbon atoms on the deoxyribose sugar. The phosphate group connects the 5' carbon of one sugar to the 3' carbon of the next, forming the sugar-phosphate backbone.
This antiparallel arrangement is crucial for the stability and functionality of the double helix. It allows for the specific pairing of bases through hydrogen bonds.
Base Pairing: The Specificity of A-T and G-C
The nitrogenous bases on the two antiparallel strands don't pair randomly. They adhere to the principle of complementary base pairing, discovered by Watson and Crick:
- Adenine (A) always pairs with thymine (T). They are connected by two hydrogen bonds.
- Guanine (G) always pairs with cytosine (C). They are connected by three hydrogen bonds.
This specific pairing is essential for several reasons:
- Maintaining the double helix structure: The hydrogen bonds between the bases hold the two strands together, stabilizing the double helix.
- Accurate DNA replication: During replication, the two strands separate, and each strand serves as a template for the synthesis of a new complementary strand. The specific base pairing ensures that the new strands are accurate copies of the originals.
- Precise gene expression: The sequence of bases dictates the sequence of amino acids in proteins. The accuracy of base pairing is crucial for the correct synthesis of proteins.
The Major and Minor Grooves: Accessibility for Proteins
The double helix is not a uniform cylinder; it has two grooves of differing widths: the major groove and the minor groove. These grooves are crucial because they provide access points for proteins that interact with DNA.
- Major groove: This wider groove allows more extensive contact between proteins and the DNA bases. Many proteins, including transcription factors, recognize and bind to specific DNA sequences in the major groove.
- Minor groove: This narrower groove provides less extensive contact, but it still plays a role in protein-DNA interactions.
DNA Supercoiling: Compacting the Genetic Material
A typical human cell contains meters of DNA packed into a tiny nucleus. This is achieved through a process called supercoiling. The double helix is further twisted and coiled upon itself, forming a compact structure called chromatin. The level of supercoiling is carefully regulated and is essential for gene expression and DNA replication. Changes in supercoiling can affect the accessibility of DNA to proteins and influence the expression of genes.
The Role of Strands in DNA Replication
The antiparallel nature of the DNA strands is critical during DNA replication. The enzyme DNA polymerase can only add nucleotides to the 3' end of a growing strand. This leads to the formation of a leading strand (synthesized continuously) and a lagging strand (synthesized discontinuously in short fragments called Okazaki fragments). Both strands, however, are crucial for producing two identical copies of the original DNA molecule, faithfully preserving the genetic information.
DNA Damage and Repair: Maintaining Genomic Integrity
DNA is constantly exposed to damaging agents, both internal and external. These agents can cause various types of DNA damage, including single-strand breaks, double-strand breaks, and base modifications. The integrity of both strands is essential for the cell's survival. Cells have developed intricate DNA repair mechanisms to correct these damages and maintain the integrity of the genome. These repair pathways often involve the coordinated action of multiple proteins that recognize, process, and repair the damaged DNA strands. Failure in these repair mechanisms can lead to mutations and potentially contribute to diseases like cancer.
Beyond the Double Helix: Alternative DNA Structures
While the canonical B-DNA double helix is the most prevalent form of DNA, other structures exist under specific conditions. These include A-DNA (a more compact form), Z-DNA (a left-handed helix), and various other non-B DNA structures like cruciforms and hairpins. These alternative structures often play important roles in gene regulation and can be influenced by the specific sequence of the DNA strands and the surrounding environment. Understanding these alternative structures is increasingly important for comprehending complex cellular processes and diseases.
Conclusion: The Intricate Dance of DNA Strands
The two strands of the DNA double helix are not merely paired entities; they are intricately interwoven components that drive the fundamental processes of life. Their antiparallel nature, specific base pairing, and interaction with proteins dictate the structure, stability, and functionality of DNA. Understanding the intricacies of DNA strands is essential not just for appreciating the elegance of the molecule but also for comprehending the mechanisms of heredity, gene expression, and disease pathogenesis. Ongoing research continues to reveal the deeper secrets held within these seemingly simple strands, highlighting the ongoing importance of studying this fundamental molecule of life. Further exploration into the dynamic interactions of these strands will undoubtedly lead to future advancements in biotechnology, medicine, and our understanding of life itself.
Latest Posts
Latest Posts
-
Describe The Organization Of The Eukaryotic Chromosome
May 10, 2025
-
Based On A Countrys Aggregate Production Function
May 10, 2025
-
Constant And Variable Regions Of Antibodies
May 10, 2025
-
Lactose Is A Disaccharide Formed By The Formation Of A
May 10, 2025
-
Curved Arrows Are Used To Illustrate
May 10, 2025
Related Post
Thank you for visiting our website which covers about The Strands In A Double Helix Of Dna Are . We hope the information provided has been useful to you. Feel free to contact us if you have any questions or need further assistance. See you next time and don't miss to bookmark.