The Types Of Bonds Found In Nucleic Acids Are
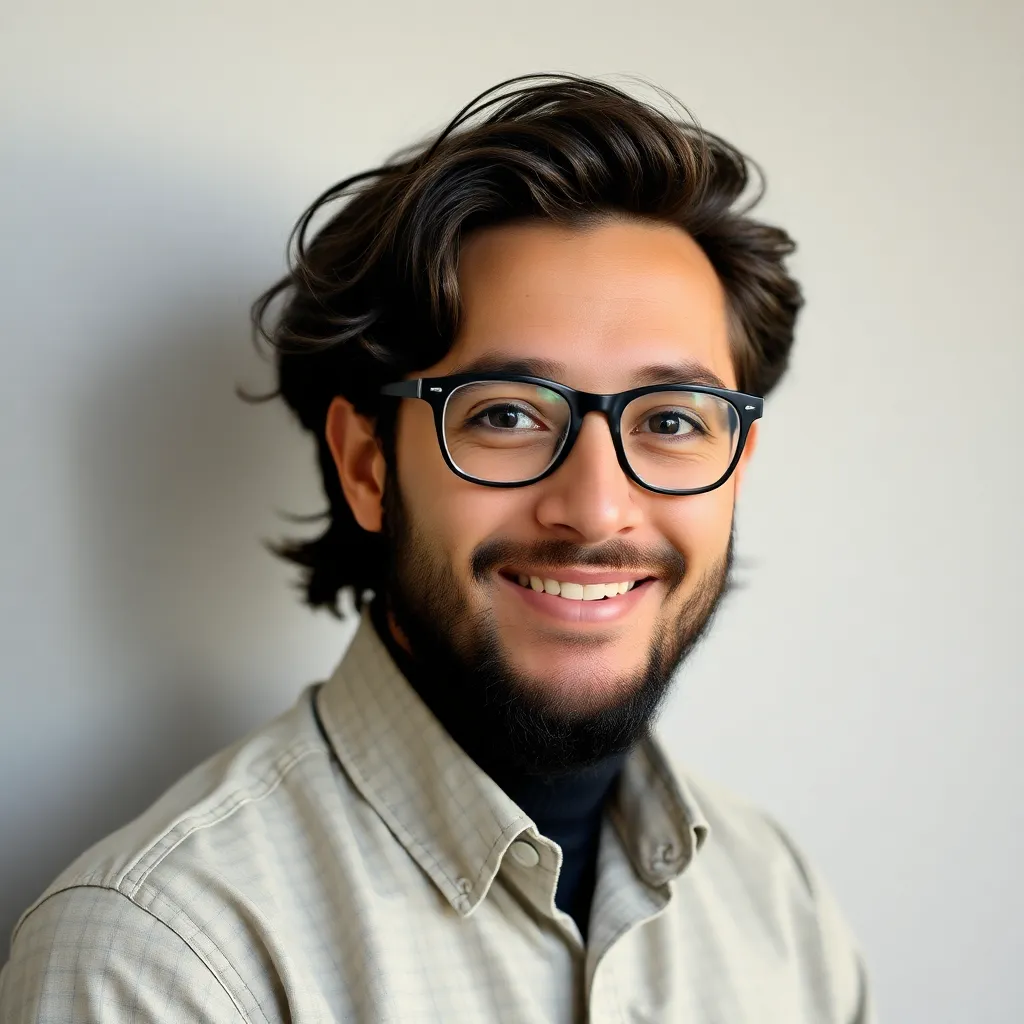
Muz Play
Apr 18, 2025 · 7 min read

Table of Contents
The Types of Bonds Found in Nucleic Acids Are...A Deep Dive
Nucleic acids, the fundamental building blocks of life, are complex macromolecules responsible for storing and transmitting genetic information. Understanding the types of bonds that hold these molecules together is crucial to comprehending their structure, function, and overall biological significance. This article will delve into the various bonds within nucleic acids, exploring their properties and roles in the intricate world of molecular biology.
The Primary Bonds: Phosphodiester Bonds and the Sugar-Phosphate Backbone
The backbone of a nucleic acid strand, whether DNA (deoxyribonucleic acid) or RNA (ribonucleic acid), is formed by a series of phosphodiester bonds. These covalent bonds link the 3'-carbon atom of one sugar molecule to the 5'-carbon atom of the next sugar molecule via a phosphate group. This linkage creates a directional polarity to the nucleic acid chain, with a 5' end (possessing a free 5'-phosphate group) and a 3' end (possessing a free 3'-hydroxyl group).
Understanding the Phosphodiester Bond Formation
The formation of a phosphodiester bond involves a dehydration reaction. A hydroxyl group (-OH) from the 3'-carbon of one sugar and a hydroxyl group from the phosphate group react, releasing a water molecule and forming a new covalent bond. This bond is relatively strong and stable under physiological conditions, contributing to the structural integrity of the nucleic acid strand. The stability is crucial because it safeguards the integrity of the genetic information encoded within the sequence.
The Role of the Sugar-Phosphate Backbone
The sugar-phosphate backbone serves several critical functions:
- Structural Support: It provides the fundamental scaffold upon which the nucleotide bases are arranged, determining the overall shape and dimensions of the DNA or RNA molecule. This structure is critical for the interactions with proteins and other molecules involved in DNA replication, transcription, and translation.
- Polarity Determination: The 5' to 3' directionality of the backbone is essential for the accurate replication and transcription of genetic information. Enzymes involved in these processes recognize and interact specifically with the 5' and 3' ends of the nucleic acid strand.
- Charge Distribution: The phosphate groups carry a negative charge at physiological pH, influencing the molecule's interaction with the environment and other charged molecules. This negative charge also contributes to the solubility of nucleic acids in water.
The Secondary Bonds: Hydrogen Bonds and Base Pairing
While phosphodiester bonds form the backbone, it's the hydrogen bonds between complementary nitrogenous bases that determine the secondary structure of nucleic acids, most notably the double helix of DNA and the various secondary structures of RNA. These relatively weak bonds are essential for the specificity and stability of base pairing and the overall three-dimensional structure of nucleic acids.
Chargaff's Rules and Base Pairing Specificity
Erwin Chargaff's rules state that in DNA, the amount of adenine (A) is equal to the amount of thymine (T), and the amount of guanine (G) is equal to the amount of cytosine (C). This equality is a direct consequence of the specific base pairing dictated by hydrogen bonding.
- Adenine (A) pairs with Thymine (T): Two hydrogen bonds form between A and T.
- Guanine (G) pairs with Cytosine (C): Three hydrogen bonds form between G and C.
In RNA, thymine is replaced by uracil (U), and the base pairing is as follows:
- Adenine (A) pairs with Uracil (U): Two hydrogen bonds form between A and U.
- Guanine (G) pairs with Cytosine (C): Three hydrogen bonds form between G and C.
The Significance of Hydrogen Bonds in Nucleic Acid Structure and Function
The hydrogen bonds between base pairs are crucial for several reasons:
- Specificity: The specific hydrogen bonding patterns ensure the accurate pairing of bases, preserving the integrity of genetic information. Errors in base pairing can lead to mutations with potentially significant consequences.
- Stability: Although individually weak, the cumulative effect of many hydrogen bonds provides significant stability to the double helix structure of DNA and the secondary structures of RNA. The number of hydrogen bonds between base pairs influences the stability of the double helix; G-C base pairs, with three hydrogen bonds, are stronger than A-T base pairs, with two hydrogen bonds.
- Dynamic Nature: The relatively weak nature of hydrogen bonds allows for the reversible separation of DNA strands during processes like DNA replication and transcription. This dynamic aspect is crucial for the biological function of nucleic acids.
- Base Stacking: While hydrogen bonding contributes significantly to base pairing, another crucial factor in stabilizing the double helix is base stacking. The planar aromatic bases stack on top of each other, leading to hydrophobic interactions that also contribute to the overall stability of the DNA double helix.
Tertiary and Quaternary Structures: Beyond the Double Helix
While hydrogen bonds define the secondary structure, DNA and RNA also exhibit tertiary and quaternary structures.
DNA Tertiary Structure: Supercoiling and Compaction
The double helix of DNA is further compacted and organized into higher-order structures. Supercoiling, a twisting of the double helix upon itself, is a common tertiary structure. This supercoiling facilitates the compact packaging of DNA within the cell nucleus. Other tertiary structures involve the interaction of DNA with proteins, such as histones, to form chromatin fibers.
RNA Tertiary Structure: Diverse Folding Patterns
RNA molecules, unlike DNA, exhibit a much greater diversity of tertiary structures. The single-stranded nature of RNA allows for extensive folding and the formation of complex three-dimensional shapes. These shapes are crucial for the specific functions of different RNA molecules, including tRNA (transfer RNA), rRNA (ribosomal RNA), and various non-coding RNAs. Hydrogen bonds, along with other interactions like base stacking, hydrophobic interactions, and sometimes metal ion interactions, contribute to the formation and stability of these complex structures.
Quaternary Structure: Multi-Subunit Complexes
In some cases, nucleic acids can form quaternary structures, where multiple individual molecules interact to form larger complexes. For instance, ribosomes, which are essential for protein synthesis, are composed of both rRNA and proteins, exhibiting a complex quaternary structure.
Other Important Interactions in Nucleic Acid Structure
Beyond the primary hydrogen and phosphodiester bonds, other interactions also play a role in stabilizing nucleic acid structures:
- Hydrophobic Interactions: Base stacking within the DNA double helix is largely driven by hydrophobic interactions between the relatively nonpolar aromatic bases. These interactions contribute significantly to the overall stability of the structure.
- Ionic Interactions: The negatively charged phosphate backbone of nucleic acids can interact with positively charged ions, such as magnesium ions (Mg²⁺), neutralizing the charge and contributing to stability. These interactions are particularly crucial in stabilizing the structure of the DNA double helix and RNA tertiary structures.
- Van der Waals Forces: These weak, short-range forces also contribute to the overall stability of nucleic acid structures, particularly in base stacking interactions.
The Importance of Understanding Nucleic Acid Bonding
Understanding the types of bonds present in nucleic acids is essential for several reasons:
- Molecular Biology Research: Research in areas such as DNA replication, transcription, translation, and gene regulation critically depends on the understanding of nucleic acid structure and the interactions between different molecules.
- Drug Design: Many drugs target nucleic acids, either directly or indirectly, and understanding the interactions between these drugs and nucleic acids is crucial for designing effective therapeutic agents.
- Genetic Engineering: Techniques such as PCR (Polymerase Chain Reaction) and gene editing rely on a detailed understanding of nucleic acid structure and properties.
- Forensic Science: DNA fingerprinting and other forensic applications rely on the ability to analyze and manipulate DNA molecules, requiring a thorough understanding of nucleic acid bonding.
In conclusion, the structure and function of nucleic acids are intricately linked to the various types of bonds that hold them together. From the strong covalent phosphodiester bonds forming the backbone to the weaker hydrogen bonds crucial for base pairing and higher-order structures, the interplay of these bonds creates the remarkable molecules that underpin all life on Earth. A deeper understanding of these bonds continues to be a crucial area of research in many scientific disciplines.
Latest Posts
Latest Posts
-
According To Bronsted Lowry Theory An Acid Is
Apr 19, 2025
-
Does The Entropy Of The Surroundings Increase For Spontaneous Processes
Apr 19, 2025
-
Construct An Mo Diagram For The He 2 Ion
Apr 19, 2025
-
Can The Zero Vector Be An Eigenvector
Apr 19, 2025
-
Concept Map Blood Groups And Transfusions
Apr 19, 2025
Related Post
Thank you for visiting our website which covers about The Types Of Bonds Found In Nucleic Acids Are . We hope the information provided has been useful to you. Feel free to contact us if you have any questions or need further assistance. See you next time and don't miss to bookmark.