Under What Circumstances Can Energy Level Transitions Occur
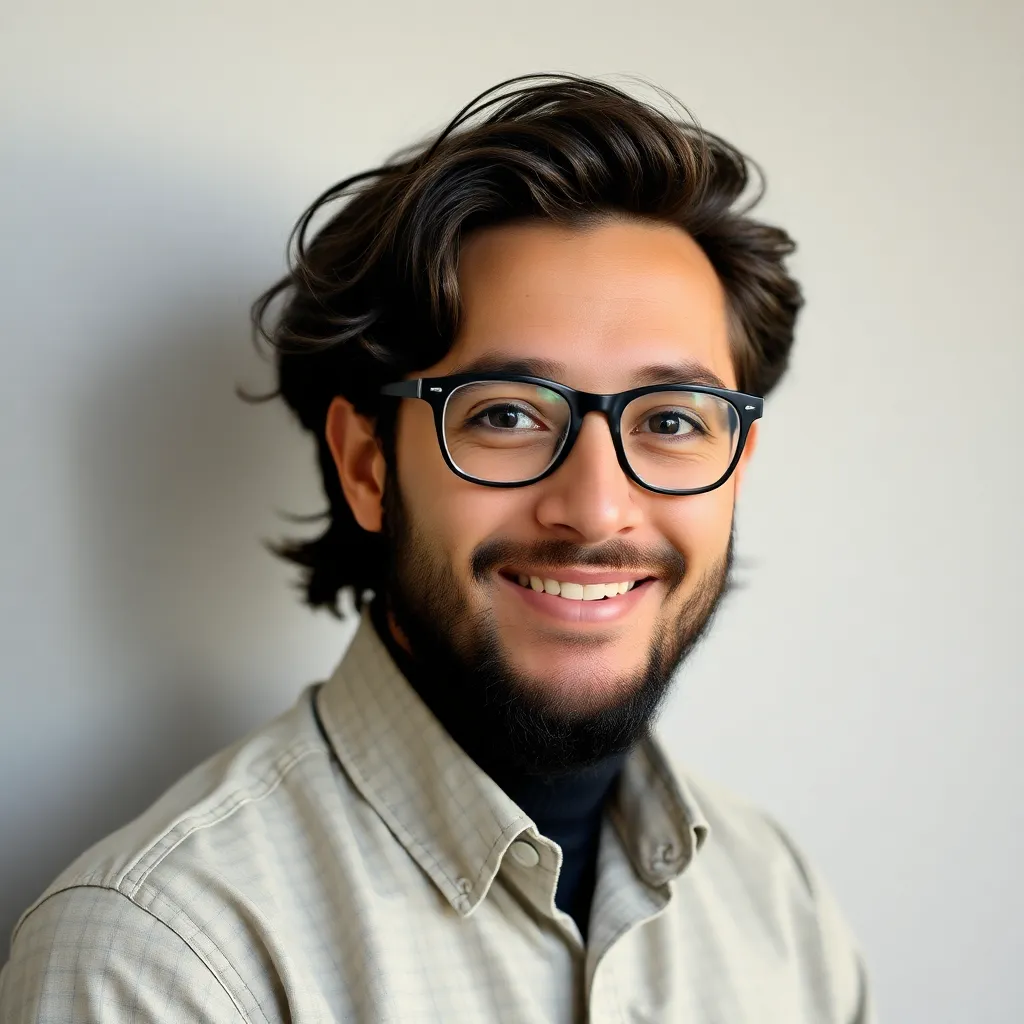
Muz Play
Apr 27, 2025 · 6 min read

Table of Contents
Under What Circumstances Can Energy Level Transitions Occur?
Energy level transitions, the shifts of electrons between different energy levels within an atom or molecule, are fundamental processes governing the interaction of matter with light and other forms of electromagnetic radiation. Understanding the circumstances under which these transitions occur is crucial in various fields, from spectroscopy and astrophysics to materials science and quantum computing. This article will delve into the intricate details of these transitions, exploring the underlying mechanisms and the diverse factors that influence them.
The Quantum Mechanical Basis of Energy Level Transitions
At the heart of energy level transitions lies the quantum mechanical description of atoms and molecules. Electrons don't occupy arbitrary energy levels but exist in specific, quantized energy states. These states are characterized by quantum numbers, which define properties like the electron's energy, angular momentum, and spin. The transition between energy levels is not a continuous process; it's a quantum leap, an instantaneous jump from one allowed energy state to another. This jump is governed by the fundamental laws of quantum mechanics, specifically the time-dependent Schrödinger equation.
Absorption and Emission of Photons
The most common way energy level transitions occur is through the absorption or emission of photons. A photon is a quantum of light, carrying energy proportional to its frequency (E = hf, where h is Planck's constant and f is the frequency).
-
Absorption: When an atom or molecule absorbs a photon, the electron jumps to a higher energy level. This is only possible if the photon's energy precisely matches the energy difference between the initial and final energy levels. This precise energy matching is a cornerstone of atomic spectroscopy, allowing us to identify elements based on their characteristic absorption spectra. The absorbed photon's energy is transferred to the electron, increasing its potential energy.
-
Emission: Conversely, when an electron in a higher energy level transitions to a lower energy level, it emits a photon. The emitted photon's energy is equal to the energy difference between the two levels. This process is responsible for the emission spectra observed in various phenomena like fluorescence, phosphorescence, and the light emitted by stars. The excess energy of the electron is converted into the energy of the emitted photon.
Selection Rules: Not All Transitions Are Allowed
While the energy matching is a necessary condition for a transition, it's not sufficient. Selection rules, derived from quantum mechanics, dictate which transitions are allowed and which are forbidden (or highly improbable). These rules depend on the changes in the quantum numbers of the electron during the transition. For instance, transitions involving changes in the electron's angular momentum are governed by specific rules, often leading to some transitions being much weaker or completely absent in the observed spectrum. These selection rules simplify the complexity of possible transitions, making spectral analysis more manageable.
Factors Influencing Energy Level Transitions
Several factors can influence the likelihood and rate of energy level transitions:
1. The Strength of the Electromagnetic Field
The intensity of the electromagnetic field plays a crucial role. A stronger field leads to a higher probability of absorption or stimulated emission. Stimulated emission is a crucial principle behind lasers, where a strong electromagnetic field triggers the emission of photons with the same properties as the incident photons.
2. Temperature
Temperature significantly impacts the population of electrons in different energy levels. At higher temperatures, more electrons occupy higher energy levels, leading to a greater probability of transitions from these levels. This is reflected in the broadening and shifting of spectral lines at higher temperatures. The thermal energy influences the distribution of electrons according to the Boltzmann distribution.
3. Pressure
Pressure affects the energy levels themselves through interatomic or intermolecular interactions. At higher pressures, these interactions broaden and shift the energy levels, influencing the absorption and emission spectra. This pressure broadening is particularly relevant in astrophysical studies where high densities of matter exist.
4. External Fields (Electric and Magnetic)
External electric and magnetic fields can significantly affect energy levels and, consequently, transitions. The Stark effect describes the splitting of energy levels in the presence of an electric field, while the Zeeman effect describes the splitting in a magnetic field. These effects modify the selection rules and lead to changes in the observed spectra, offering valuable information about the structure of atoms and molecules.
5. Interaction with Other Particles
Collisions with other particles (atoms, molecules, or ions) can induce transitions. These collisional processes can lead to energy transfer, causing an electron to jump to a different energy level. This is particularly important in plasmas where frequent collisions occur. The rate of these collisional transitions depends on the density and temperature of the medium.
Specific Examples of Energy Level Transitions
Let's examine specific examples where energy level transitions play a crucial role:
1. Atomic Spectroscopy: Identifying Elements
Atomic spectroscopy relies heavily on the unique energy level transitions of each element. When an element is heated or excited electrically, its atoms emit photons corresponding to specific energy level transitions. The resulting emission spectrum is a characteristic "fingerprint" of the element, allowing for its identification. This principle is widely used in analytical chemistry and astronomy.
2. Molecular Spectroscopy: Studying Molecular Structure and Dynamics
Molecular spectroscopy uses similar principles to study the structure and dynamics of molecules. Molecules have more complex energy levels than atoms due to vibrational and rotational motions in addition to electronic transitions. Analyzing the absorption and emission spectra of molecules reveals details about their bond lengths, bond angles, and vibrational frequencies. This information is invaluable in understanding chemical reactions and molecular properties.
3. Lasers: Stimulated Emission for Coherent Light
Lasers (Light Amplification by Stimulated Emission of Radiation) utilize stimulated emission to produce highly coherent and monochromatic light. A population inversion, where more electrons occupy a higher energy level than a lower one, is created. When a photon with the correct energy interacts with an excited electron, it triggers the emission of an identical photon, leading to amplification of the light.
4. Astrophysics: Studying Stars and Nebulae
The light from stars and nebulae provides valuable information about their composition and physical conditions. By analyzing the spectral lines, astronomers can determine the elemental abundances, temperatures, densities, and velocities of celestial objects. Energy level transitions are essential for understanding stellar nucleosynthesis and the evolution of stars.
5. Quantum Computing: Utilizing Quantum Superpositions
Quantum computing exploits the quantum properties of atoms and molecules, including energy level transitions. Quantum bits (qubits) can be encoded in the different energy levels of a system, and controlled transitions between these levels allow for quantum operations, which are the basis of quantum computation. The manipulation of energy levels is central to building quantum computers.
Conclusion: A Dynamic and Versatile Phenomenon
Energy level transitions are fundamental processes governing the interaction of matter with light and other forms of energy. The circumstances under which these transitions occur are governed by the principles of quantum mechanics, with various factors such as electromagnetic fields, temperature, pressure, and external fields influencing the likelihood and rate of transitions. Understanding these processes is crucial for advancements in various fields, from spectroscopy and astrophysics to materials science and quantum computing. The precise nature of these transitions continues to be a subject of active research, pushing the boundaries of our understanding of the quantum world and its impact on the macroscopic world. The study of energy level transitions remains a vibrant and critical area of scientific inquiry, constantly revealing new insights into the fundamental laws of nature.
Latest Posts
Latest Posts
-
How To Derive A Demand Function From A Utility Function
Apr 27, 2025
-
Retention Is How The Brain Encodes Information
Apr 27, 2025
-
Journal Entry For Notes Payable With Interest
Apr 27, 2025
-
How To Find The Minimum And Maximum Of A Graph
Apr 27, 2025
-
A Polygon With Three Sides And One Right Angle
Apr 27, 2025
Related Post
Thank you for visiting our website which covers about Under What Circumstances Can Energy Level Transitions Occur . We hope the information provided has been useful to you. Feel free to contact us if you have any questions or need further assistance. See you next time and don't miss to bookmark.