Using Models To Predict Molecular Structure Lab
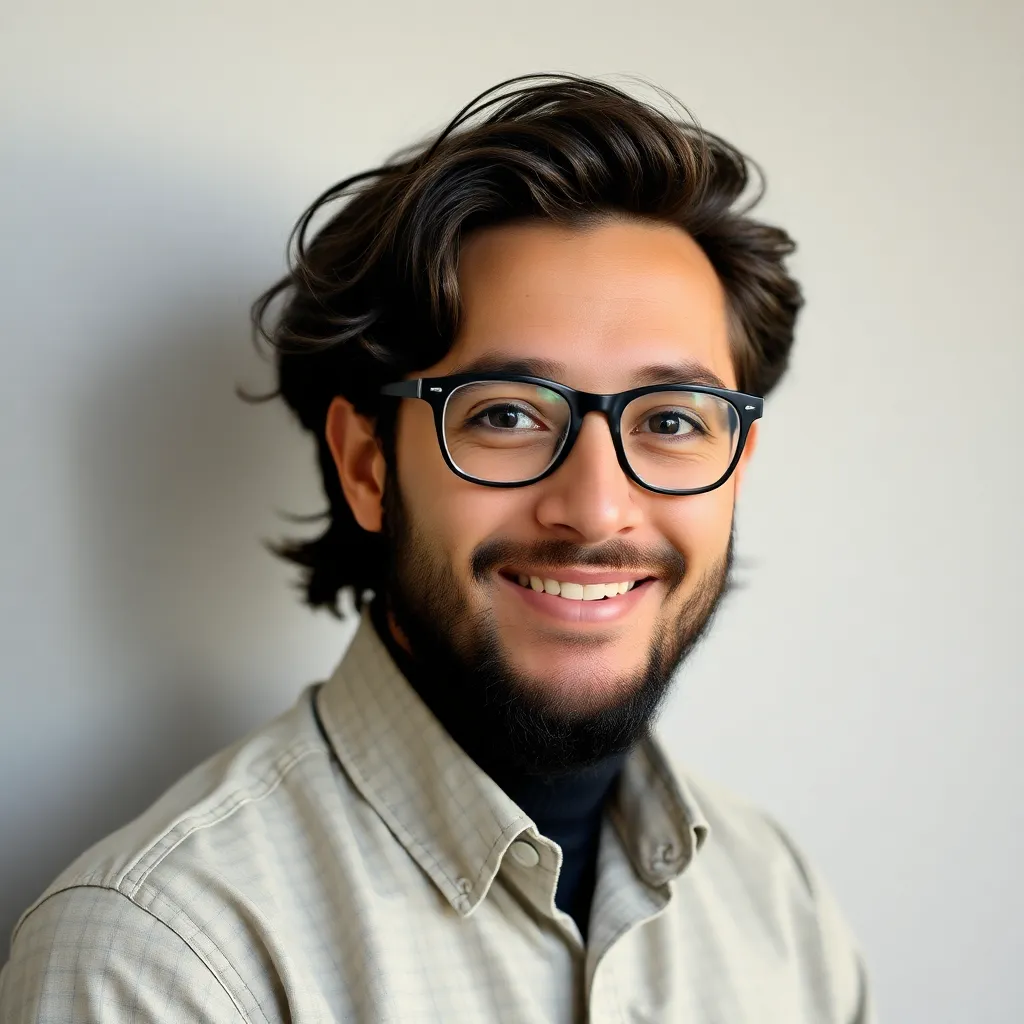
Muz Play
Mar 12, 2025 · 5 min read

Table of Contents
Using Models to Predict Molecular Structure: A Comprehensive Guide for Lab Experiments
Predicting molecular structure is a cornerstone of modern chemistry, impacting various fields from drug discovery to materials science. While traditional experimental techniques remain crucial, computational modeling offers a powerful complement, accelerating research and providing insights inaccessible through experimentation alone. This article delves into the practical application of computational models in predicting molecular structure within a laboratory setting, covering key aspects from choosing the right model to interpreting the results and their limitations.
Understanding the Power of Computational Models in Predicting Molecular Structure
Experimental determination of molecular structure, methods like X-ray crystallography and NMR spectroscopy, can be time-consuming, expensive, and sometimes impossible for certain molecules. Computational modeling provides a cost-effective and rapid alternative, allowing researchers to:
1. Predict Structures Before Synthesis:
Modeling allows researchers to predict the likely structure of a molecule before attempting its synthesis. This can save significant time and resources by identifying potentially unstable or challenging-to-synthesize structures early in the process.
2. Explore Conformational Landscapes:
Many molecules exhibit multiple stable conformations (different arrangements of atoms in space). Computational models can effectively explore this conformational landscape, identifying the most stable and relevant conformers for a given molecule under specific conditions (temperature, solvent, etc.). This is critical for understanding a molecule's reactivity and properties.
3. Study Reactive Intermediates:
Some molecular species are highly reactive and short-lived, making their experimental characterization difficult. Computational modeling can be used to predict the structure and properties of these reactive intermediates, providing valuable insights into reaction mechanisms.
4. Analyze Large and Complex Molecules:
Modeling becomes increasingly valuable when dealing with large biomolecules (proteins, nucleic acids) or complex materials, where experimental structure determination can be extremely challenging.
Choosing the Right Computational Model
The choice of computational model depends heavily on the size and complexity of the molecule, the desired level of accuracy, and the available computational resources. Several key models are widely used:
1. Molecular Mechanics (MM):
MM methods employ classical mechanics principles, representing atoms as spheres with defined forces acting between them. These methods are computationally inexpensive and suitable for studying large systems, but lack the accuracy of quantum mechanical methods for describing electronic interactions. Suitable for: large molecules, conformational analysis, molecular dynamics simulations.
2. Semi-empirical Methods:
Semi-empirical methods are a compromise between MM and ab initio quantum mechanical methods. They incorporate some quantum mechanical principles but utilize parameters derived from experimental data to simplify calculations. Suitable for: medium-sized molecules, where higher accuracy than MM is needed but ab initio methods are computationally prohibitive. Examples include AM1, PM3, and MNDO.
3. Ab Initio Methods:
Ab initio methods solve the Schrödinger equation directly without empirical parameters. They provide high accuracy but are computationally expensive, limiting their application to smaller molecules. Suitable for: high-accuracy structure prediction, detailed analysis of electronic properties. Examples include Hartree-Fock (HF) and various post-HF methods like MP2, CCSD, and coupled-cluster methods.
4. Density Functional Theory (DFT):
DFT is a widely used quantum mechanical method that focuses on the electron density rather than the wavefunction. It offers a good balance between accuracy and computational cost, making it suitable for a broad range of molecules and systems. Suitable for: medium to large molecules, where high accuracy is required. Popular DFT functionals include B3LYP, PBE, and M06-2X.
Practical Steps in Using Models to Predict Molecular Structure in a Lab Setting
The process of using computational models to predict molecular structure typically involves these steps:
1. Building the Molecular Model:
This involves using molecular modeling software (e.g., Gaussian, Spartan, Avogadro) to construct a three-dimensional representation of the molecule based on its chemical formula and connectivity. Care must be taken to ensure proper bond lengths, angles, and dihedral angles.
2. Geometry Optimization:
The initial molecular model is rarely the true minimum energy structure. Geometry optimization algorithms are employed to refine the structure by minimizing the energy of the molecule. This is achieved by iteratively adjusting the atomic positions until a stable, low-energy structure is found.
3. Frequency Calculation:
After geometry optimization, a frequency calculation is often performed. This confirms that the optimized structure is a true minimum (no imaginary frequencies) and provides vibrational frequencies, which can be compared with experimental data (IR and Raman spectroscopy).
4. Analyzing the Results:
The results of the calculation include the optimized molecular structure (bond lengths, angles, dihedrals), energy, vibrational frequencies, and various other properties. These data are analyzed to assess the validity of the model and draw conclusions about the molecular structure.
5. Comparing with Experimental Data:
When experimental data (e.g., NMR, X-ray crystallography) is available, the computational results should be compared to assess the accuracy of the model. Discrepancies may indicate limitations of the model or errors in the experimental data.
Interpreting Results and Addressing Limitations
It’s crucial to understand the limitations of computational models:
- Approximations: All computational models rely on approximations, and the accuracy of the prediction depends on the level of theory and the chosen basis set.
- Computational Cost: Higher levels of theory provide greater accuracy but are computationally more expensive. A balance must be struck between accuracy and feasibility.
- Solvent Effects: Many reactions and molecular properties are influenced by the solvent. Explicitly modeling the solvent molecules significantly increases the computational cost. Implicit solvent models are often used as a compromise.
- Dynamic Effects: Molecular structures are not static; they are constantly vibrating and moving. Molecular dynamics simulations can address this, but they are computationally demanding.
Conclusion: Integrating Models into Your Lab Workflow
Computational modeling offers a powerful tool for predicting molecular structure, complementing traditional experimental techniques. By carefully selecting the appropriate model, performing rigorous calculations, and critically evaluating the results, researchers can gain valuable insights into molecular structure, reactivity, and properties, significantly accelerating their research and leading to more efficient and effective experimentation. The integration of computational modeling into the lab workflow represents a significant advancement in modern chemistry, unlocking new possibilities for discovery and innovation. Remember that computational modeling should be viewed as a powerful tool to complement, not replace, experimental investigations. The synergy between computation and experiment is key to achieving accurate and reliable results.
Latest Posts
Latest Posts
-
Are Moles Conserved In A Chemical Reaction
May 09, 2025
-
What Makes A Cell A Target Cell For A Hormone
May 09, 2025
-
As Nutritional Energy Passes Through The Food Chain Energy
May 09, 2025
-
What Is The N Type Semiconductor
May 09, 2025
-
How Do Objects Become Negatively Charged
May 09, 2025
Related Post
Thank you for visiting our website which covers about Using Models To Predict Molecular Structure Lab . We hope the information provided has been useful to you. Feel free to contact us if you have any questions or need further assistance. See you next time and don't miss to bookmark.