What Are The Reactants Of The Electron Transport Chain
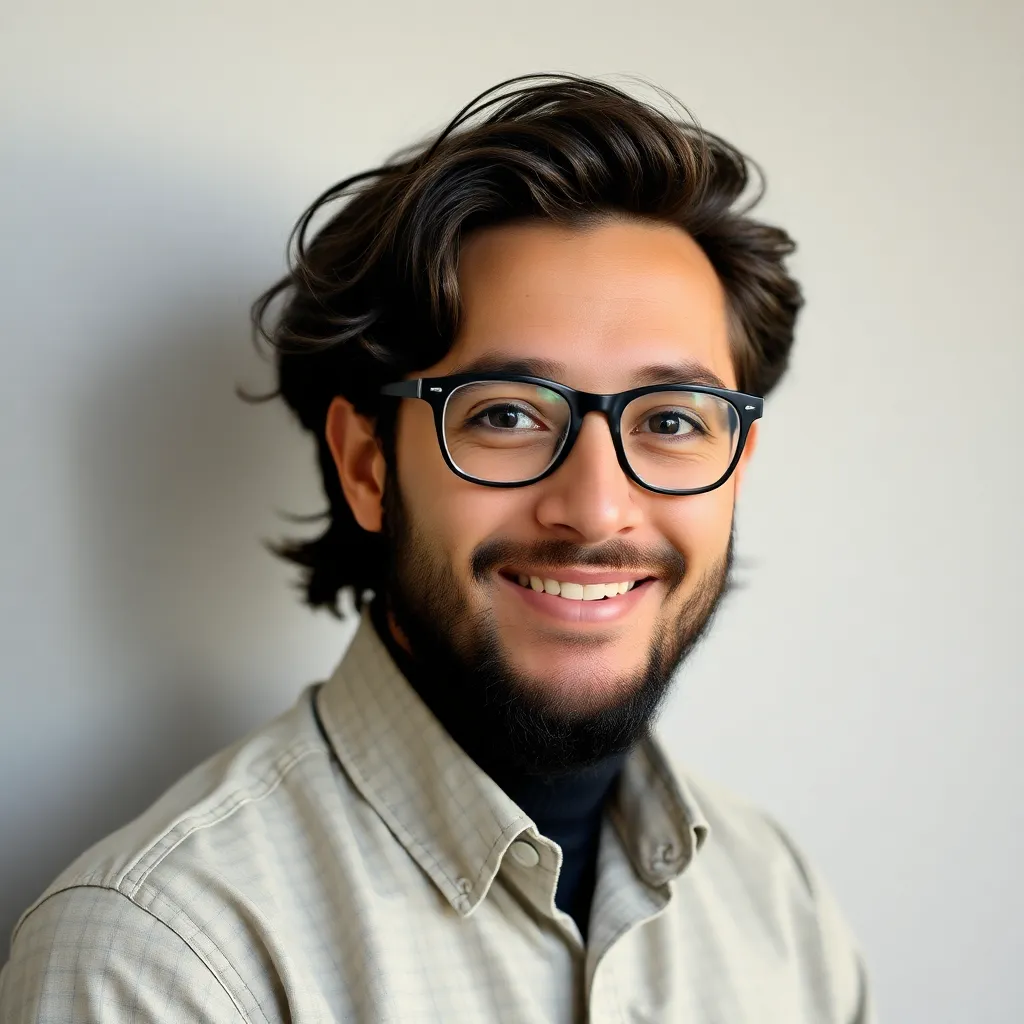
Muz Play
May 11, 2025 · 5 min read
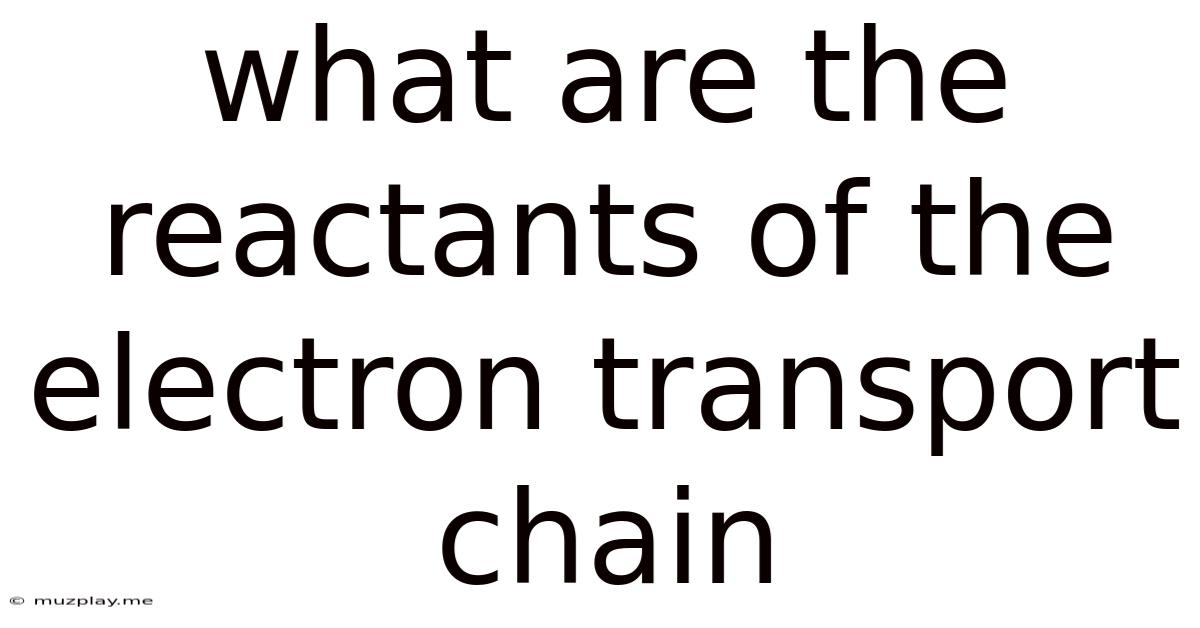
Table of Contents
What Are the Reactants of the Electron Transport Chain?
The electron transport chain (ETC), also known as the respiratory chain, is a series of protein complexes embedded in the inner mitochondrial membrane of eukaryotic cells and the plasma membrane of prokaryotic cells. It plays a crucial role in cellular respiration, the process by which cells generate energy in the form of ATP (adenosine triphosphate). Understanding the reactants of the ETC is essential to grasping the intricate mechanics of this vital energy-producing pathway. This article will delve deep into the specific reactants, their roles, and the overall process, providing a comprehensive overview for students and researchers alike.
The Primary Reactants: NADH and FADH₂
The primary reactants of the electron transport chain are NADH (nicotinamide adenine dinucleotide) and FADH₂ (flavin adenine dinucleotide). These two molecules are crucial electron carriers, generated during earlier stages of cellular respiration: glycolysis and the citric acid cycle (also known as the Krebs cycle or TCA cycle).
NADH: The High-Energy Electron Carrier
NADH is produced during glycolysis and the citric acid cycle. It carries a high-energy electron pair, acquired by the reduction of NAD⁺ (the oxidized form of NADH). This reduction occurs when NAD⁺ accepts two electrons and a proton (H⁺), effectively storing the energy released from the oxidation of glucose and other fuel molecules. The high-energy electrons in NADH are then subsequently donated to the ETC, initiating the process of oxidative phosphorylation.
FADH₂: A Slightly Less Energetic Electron Carrier
Similar to NADH, FADH₂ is also a crucial electron carrier generated during the citric acid cycle. However, FADH₂ carries a slightly lower energy electron pair compared to NADH. This difference in energy levels directly impacts the amount of ATP produced per molecule. FADH₂ donates its electrons to a different point in the ETC than NADH, resulting in a smaller proton gradient and thus less ATP synthesis.
The Role of Oxygen: The Terminal Electron Acceptor
While NADH and FADH₂ provide the electrons, the electron transport chain also necessitates a terminal electron acceptor. This crucial role is fulfilled by molecular oxygen (O₂). Oxygen's high electronegativity makes it an ideal final recipient for the electrons traveling down the ETC.
The Reduction of Oxygen to Water
As electrons move down the ETC, their energy is progressively harnessed to pump protons (H⁺) across the inner mitochondrial membrane, establishing a proton gradient. Finally, these electrons reach complex IV (cytochrome c oxidase), where they are transferred to molecular oxygen. This process reduces oxygen, combining it with protons to form water (H₂O). This reaction is essential for the continuous operation of the ETC, as without the terminal electron acceptor, the chain would become blocked, halting ATP production.
The Electron Transport Chain Complexes: Facilitating Electron Transfer
The electron transport chain isn't simply a linear pathway; it comprises four major protein complexes (I-IV), embedded within the inner mitochondrial membrane, along with two mobile electron carriers: ubiquinone (Q, also known as coenzyme Q) and cytochrome c. Each complex plays a vital role in facilitating electron transfer and proton pumping.
Complex I (NADH-ubiquinone oxidoreductase): Accepting Electrons from NADH
Complex I accepts electrons from NADH and transfers them to ubiquinone (Q). This transfer is coupled to the pumping of protons across the inner mitochondrial membrane, contributing to the proton gradient crucial for ATP synthesis.
Complex II (Succinate dehydrogenase): An Alternative Entry Point for Electrons
Complex II, also known as succinate dehydrogenase, is part of both the citric acid cycle and the ETC. It accepts electrons directly from FADH₂ (generated during the citric acid cycle) and transfers them to ubiquinone (Q). Importantly, complex II does not pump protons across the membrane, explaining the lower ATP yield from FADH₂.
Ubiquinone (Q): A Mobile Electron Carrier
Ubiquinone (Q) is a lipid-soluble molecule that acts as a mobile electron carrier, shuttling electrons between complex I or II and complex III.
Complex III (Ubiquinol-cytochrome c oxidoreductase): Further Electron Transfer and Proton Pumping
Complex III accepts electrons from ubiquinol (QH₂, the reduced form of Q) and transfers them to cytochrome c. This transfer is also coupled to proton pumping, further contributing to the proton gradient.
Cytochrome c: Another Mobile Electron Carrier
Cytochrome c is a water-soluble protein that acts as a mobile electron carrier, shuttling electrons between complex III and complex IV.
Complex IV (Cytochrome c oxidase): The Final Destination
Complex IV, also known as cytochrome c oxidase, accepts electrons from cytochrome c and transfers them to molecular oxygen, the terminal electron acceptor, reducing it to water. This process also contributes to proton pumping, although less significantly than complexes I and III.
The Proton Gradient and ATP Synthesis: The Final Outcome
The sequential transfer of electrons through the ETC complexes is coupled to the pumping of protons (H⁺) across the inner mitochondrial membrane. This creates a proton gradient, with a higher concentration of protons in the intermembrane space compared to the mitochondrial matrix. This gradient represents stored energy, which is harnessed by ATP synthase, a molecular rotary engine, to produce ATP from ADP and inorganic phosphate (Pi). This process is called chemiosmosis.
Other Reactants and Factors Affecting the ETC
While NADH, FADH₂, and oxygen are the primary reactants, several other factors influence the electron transport chain's efficiency:
-
pH: The pH gradient across the inner mitochondrial membrane is crucial for ATP synthesis. Changes in pH can affect the proton motive force and consequently ATP production.
-
Temperature: Temperature affects the rate of enzymatic reactions, including those involved in the ETC. Optimal temperatures are necessary for efficient functioning.
-
Inhibitors and Uncouplers: Certain molecules can inhibit the ETC by binding to specific complexes, blocking electron flow. Uncouplers disrupt the proton gradient, preventing ATP synthesis even if electron transport continues.
-
Substrate Availability: The availability of NADH and FADH₂ dictates the rate of electron transport. Reduced substrate availability will limit the ETC's activity.
Conclusion: A Complex and Crucial Process
The electron transport chain is a remarkably complex yet elegantly designed process, vital for cellular energy production. Understanding its reactants—NADH, FADH₂, and oxygen—and the intricate interplay between the complexes and electron carriers is essential to appreciating the efficiency and sophistication of cellular respiration. This intricate system, subject to various influencing factors, represents a crucial area of study in biochemistry and cellular biology, with implications for various aspects of human health and disease. Further research continues to unveil finer details of this fundamental process, revealing ever more fascinating aspects of its mechanism and regulation.
Latest Posts
Latest Posts
-
Delta G And Delta G Not
May 12, 2025
-
Electric Field Inside Parallel Plate Capacitor
May 12, 2025
-
Conditions For A Matrix To Be Diagonalizable
May 12, 2025
-
Identifying Phase Transitions On A Heating Curve
May 12, 2025
-
Is A Function Differentiable At A Vertical Tangent
May 12, 2025
Related Post
Thank you for visiting our website which covers about What Are The Reactants Of The Electron Transport Chain . We hope the information provided has been useful to you. Feel free to contact us if you have any questions or need further assistance. See you next time and don't miss to bookmark.