What Carbon Molecules Remain At The End Of Glycolysis
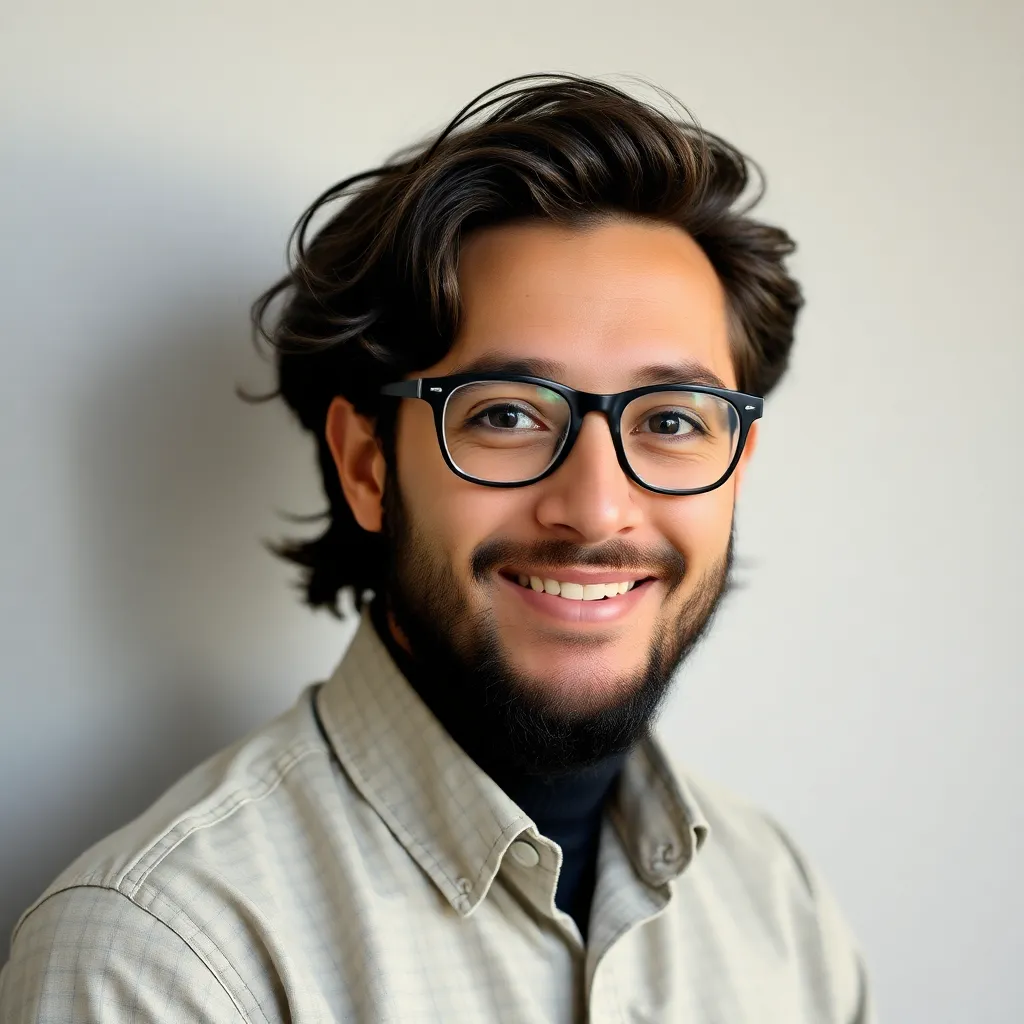
Muz Play
Apr 22, 2025 · 6 min read

Table of Contents
What Carbon Molecules Remain at the End of Glycolysis?
Glycolysis, the metabolic pathway that breaks down glucose, is a fundamental process in nearly all living organisms. Understanding its intricacies, particularly the fate of carbon molecules, is crucial for grasping cellular respiration and energy production. This article delves deep into the specifics of glycolysis, highlighting the carbon molecules that remain at its completion and explaining their subsequent roles in metabolism.
Glycolysis: A Step-by-Step Breakdown
Glycolysis, meaning "sugar splitting," is a ten-step enzymatic process that occurs in the cytoplasm of cells. It begins with a single molecule of glucose (a six-carbon sugar), and through a series of oxidation and isomerization reactions, it ultimately yields two molecules of pyruvate (a three-carbon compound). Let's examine the key steps and carbon transformations:
The Investment Phase (Steps 1-5): Priming the Pump
The initial five steps are considered the investment phase because they require energy input in the form of ATP. These steps involve phosphorylation and isomerization reactions, preparing the glucose molecule for cleavage.
- Step 1: Glucose Phosphorylation: Glucose is phosphorylated using ATP, yielding glucose-6-phosphate. This crucial step traps glucose within the cell and initiates its metabolic breakdown. The six carbons of glucose are preserved.
- Step 2: Isomerization: Glucose-6-phosphate is isomerized to fructose-6-phosphate. This rearrangement facilitates subsequent cleavage. Again, all six carbons are retained.
- Step 3: Fructose Phosphorylation: Fructose-6-phosphate is phosphorylated using another ATP molecule, producing fructose-1,6-bisphosphate. This second phosphorylation further primes the molecule for splitting. Six carbons remain intact.
- Step 4: Cleavage: Fructose-1,6-bisphosphate is cleaved into two three-carbon molecules: glyceraldehyde-3-phosphate (G3P) and dihydroxyacetone phosphate (DHAP). This is the pivotal step where the six-carbon molecule is split.
- Step 5: Isomerization: DHAP is isomerized to G3P. This ensures that both products of the cleavage are identical, setting the stage for the subsequent energy-yielding phase. Now we have two molecules of G3P, each containing three carbons.
The Payoff Phase (Steps 6-10): Energy Generation
The remaining five steps are the payoff phase, where ATP and NADH are generated. These steps involve oxidation and phosphorylation reactions, extracting energy from the G3P molecules.
- Step 6: Oxidation and Phosphorylation: G3P is oxidized, and inorganic phosphate (Pi) is added, producing 1,3-bisphosphoglycerate. This step generates NADH, a crucial electron carrier in cellular respiration. Three carbons are present in each 1,3-bisphosphoglycerate molecule.
- Step 7: Substrate-Level Phosphorylation: 1,3-bisphosphoglycerate transfers a phosphate group to ADP, generating ATP and 3-phosphoglycerate. This is the first instance of ATP production in glycolysis. Three carbons remain.
- Step 8: Isomerization: 3-phosphoglycerate is isomerized to 2-phosphoglycerate. The three carbons are rearranged, but the total number remains unchanged.
- Step 9: Dehydration: 2-phosphoglycerate undergoes dehydration, forming phosphoenolpyruvate (PEP). This reaction removes a water molecule, increasing the phosphate group's transfer potential. Three carbons are still present.
- Step 10: Substrate-Level Phosphorylation: PEP transfers its phosphate group to ADP, generating another ATP molecule and pyruvate. This is the second instance of ATP production in glycolysis. This leaves us with two molecules of pyruvate, each containing three carbon atoms.
The Fate of the Carbon Atoms: Pyruvate's Journey
The end result of glycolysis is the production of two molecules of pyruvate, each containing three carbon atoms. These three-carbon molecules represent the remaining carbons from the original six-carbon glucose molecule. The fate of these pyruvate molecules depends on the organism and the prevailing oxygen levels.
Aerobic Conditions: The Citric Acid Cycle and Oxidative Phosphorylation
Under aerobic conditions (in the presence of oxygen), pyruvate enters the mitochondria, where it undergoes further oxidation in the citric acid cycle (also known as the Krebs cycle or TCA cycle). In this cycle, the three-carbon pyruvate is completely oxidized, releasing carbon dioxide (CO2) as a byproduct. The electrons released during oxidation are passed along the electron transport chain, generating a large amount of ATP through oxidative phosphorylation. This process extracts the maximum amount of energy from glucose.
Anaerobic Conditions: Fermentation
Under anaerobic conditions (absence of oxygen), pyruvate undergoes fermentation to regenerate NAD+, a crucial coenzyme for glycolysis. Fermentation pathways vary among organisms:
- Lactic Acid Fermentation: In animals and some bacteria, pyruvate is reduced to lactate. This process regenerates NAD+ but doesn't produce any additional ATP.
- Alcoholic Fermentation: In yeast and some bacteria, pyruvate is converted to ethanol and CO2. This also regenerates NAD+ without further ATP production.
In both lactic acid and alcoholic fermentation, the carbon atoms of pyruvate are ultimately transformed into different molecules. In lactic acid fermentation, they remain in the lactate molecule. In alcoholic fermentation, one carbon atom is released as CO2, and the remaining two are incorporated into ethanol.
Regulation of Glycolysis: A Complex Balancing Act
The glycolytic pathway is tightly regulated to ensure efficient energy production and prevent wasteful expenditure of resources. Several key enzymes are regulated allosterically by metabolites such as ATP, ADP, AMP, and citrate.
- Hexokinase: Inhibited by glucose-6-phosphate, preventing excessive glucose phosphorylation when glucose-6-phosphate levels are high.
- Phosphofructokinase (PFK): The most crucial regulatory enzyme in glycolysis, it is allosterically inhibited by high levels of ATP and citrate, indicating sufficient energy reserves. It is activated by AMP and ADP, signaling low energy levels.
- Pyruvate Kinase: Inhibited by ATP and alanine, preventing excessive pyruvate formation when energy levels are high. Activated by fructose-1,6-bisphosphate, ensuring a coordinated flow through the pathway.
Beyond Glycolysis: Connecting to Other Metabolic Pathways
The products of glycolysis, particularly pyruvate and its derivatives, are crucial intermediates in numerous metabolic pathways. They feed into gluconeogenesis (glucose synthesis), lipogenesis (fatty acid synthesis), and amino acid synthesis. This intricate network of metabolic pathways ensures the efficient utilization of resources and adaptation to varying metabolic demands.
Gluconeogenesis: When glucose levels are low, pyruvate can be converted back into glucose through gluconeogenesis. This pathway reverses many of the steps in glycolysis, but it utilizes different enzymes in some key regulatory steps.
Lipogenesis: Pyruvate can be converted into acetyl-CoA, a precursor for fatty acid synthesis. Under conditions of excess energy, acetyl-CoA is utilized for the production of fatty acids, which are stored as triglycerides.
Amino Acid Synthesis: Pyruvate serves as a precursor for the synthesis of several amino acids, including alanine and aspartate. These amino acids are essential building blocks for proteins and other nitrogen-containing molecules.
Conclusion: A Central Metabolic Hub
Glycolysis is a pivotal metabolic pathway, providing the foundation for cellular energy production. The fate of the carbon atoms from glucose is intricately linked to cellular energy status and environmental conditions. The two three-carbon pyruvate molecules that remain at the end of glycolysis represent a crucial metabolic crossroads, feeding into a multitude of subsequent pathways, highlighting the central role of glycolysis in cellular metabolism and homeostasis. Understanding the intricacies of glycolysis, including the transformations of carbon atoms, is fundamental to comprehending cellular biology and metabolic regulation. Further research continues to unravel the complex interplay between glycolysis and other metabolic pathways, expanding our knowledge of cellular processes and offering new avenues for therapeutic intervention in metabolic diseases.
Latest Posts
Latest Posts
-
What Is Positive Shape In Art
Apr 22, 2025
-
Law Of Conservation Of Mass Non Examples
Apr 22, 2025
-
What Is The Function Of A Stage On A Microscope
Apr 22, 2025
-
How To Use The Ratio Test
Apr 22, 2025
-
Law Of Conservation Of Mechanical Energy Formula
Apr 22, 2025
Related Post
Thank you for visiting our website which covers about What Carbon Molecules Remain At The End Of Glycolysis . We hope the information provided has been useful to you. Feel free to contact us if you have any questions or need further assistance. See you next time and don't miss to bookmark.