What Causes Atoms To Have Magnetic Properties
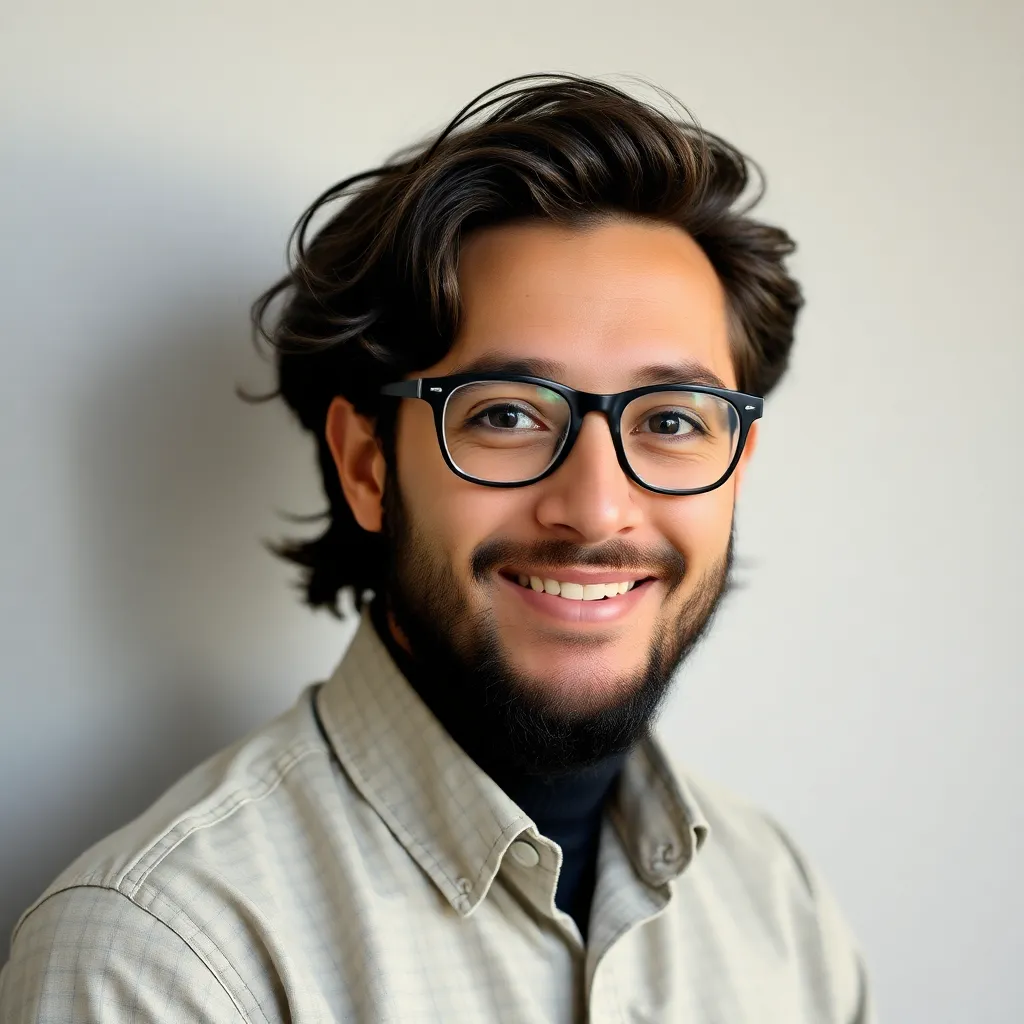
Muz Play
May 12, 2025 · 6 min read
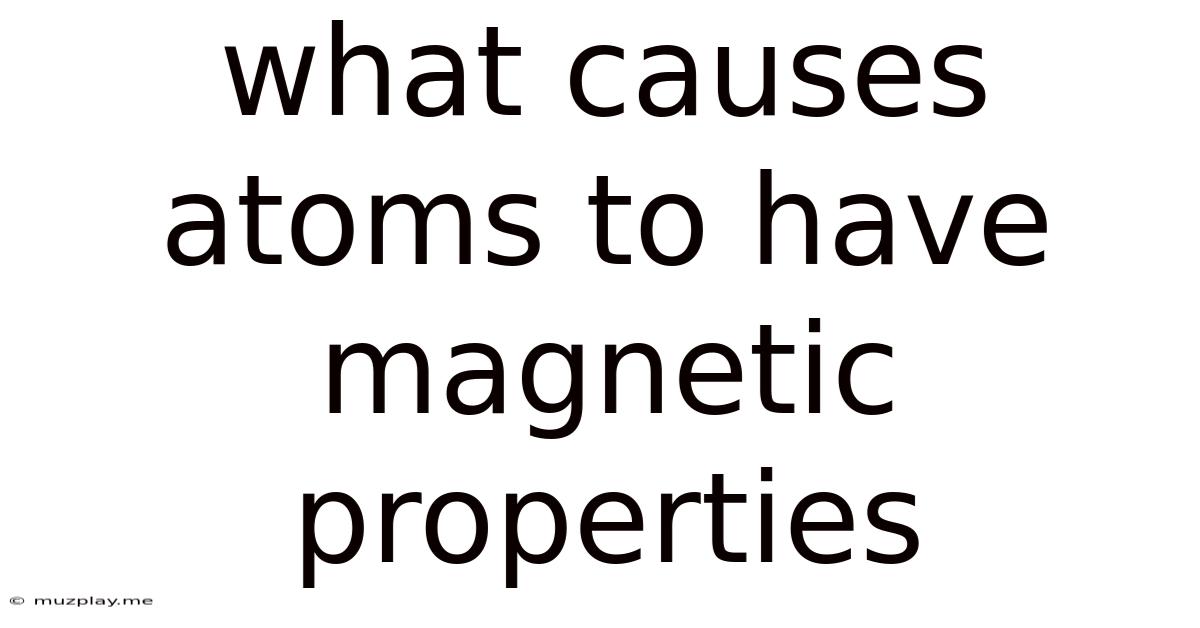
Table of Contents
What Causes Atoms to Have Magnetic Properties?
Understanding why some atoms exhibit magnetic properties while others don't delves into the fascinating world of quantum mechanics and the intricate dance of electrons within an atom. This journey begins with a foundational understanding of electron behavior and how their intrinsic properties translate to macroscopic magnetic effects.
The Electron's Dual Nature: Spin and Orbital Angular Momentum
At the heart of atomic magnetism lies the electron itself. Electrons possess two fundamental properties crucial to magnetism: spin and orbital angular momentum.
Spin Angular Momentum: An Intrinsic Property
Imagine an electron as a tiny spinning sphere (though this is a simplified analogy). This "spin" is not a literal rotation, but rather an intrinsic quantum property, much like its charge or mass. This spin is quantized, meaning it can only exist in specific, discrete states. The most important aspect for magnetism is that spin is associated with a magnetic moment, a tiny internal magnet. This magnetic moment aligns itself with an external magnetic field, contributing to the atom's overall magnetic behavior. The spin angular momentum is represented by the quantum number s, which for electrons is always ½.
Orbital Angular Momentum: Motion Around the Nucleus
Electrons don't just spin; they also orbit the nucleus. This orbital motion generates another form of angular momentum – orbital angular momentum. Similar to spin, this orbital angular momentum also creates a magnetic moment. This magnetic moment's strength is determined by the electron's orbital, described by the quantum number l, which can take values from 0 to n-1, where n is the principal quantum number defining the electron shell. The shape of the electron's orbital directly influences the magnitude of this magnetic moment. For example, s orbitals (l = 0) have spherical symmetry and zero orbital angular momentum, while p orbitals (l = 1) have a dumbbell shape and possess orbital angular momentum.
Combining Spin and Orbital Magnetic Moments: The Total Magnetic Moment
The total magnetic moment of an atom is the vector sum of the magnetic moments from all its electrons – both their spin and orbital contributions. This summation takes into account the Pauli Exclusion Principle, which dictates that no two electrons in an atom can have the same four quantum numbers (n, l, m<sub>l</sub>, m<sub>s</sub>). This principle significantly impacts the overall magnetic behavior.
Hund's Rules: Maximizing the Total Spin
Hund's rules provide guidelines for predicting the ground-state electronic configuration of an atom, particularly concerning the arrangement of electrons in subshells. These rules help determine the total spin and orbital angular momentum and thus, the overall magnetic moment. Crucially, Hund's rules state that electrons will individually occupy orbitals within a subshell before pairing up. This maximizes the total spin, leading to a stronger overall magnetic moment.
Pairing of Electrons: Cancellation of Magnetic Moments
When electrons pair up within an orbital, their individual spin magnetic moments are anti-parallel (opposite directions), resulting in a net cancellation. This is a key factor in determining whether an atom will exhibit magnetic properties. If all electron spins are paired, the atom has a net magnetic moment of zero and is diamagnetic.
Diamagnetism, Paramagnetism, and Ferromagnetism: Macroscopic Magnetic Behaviors
The total magnetic moment of an atom dictates its macroscopic magnetic behavior. There are three primary categories:
Diamagnetism: Weak Repulsion of Magnetic Fields
Diamagnetism is a fundamental property of all matter. It arises from the orbital motion of electrons. When an external magnetic field is applied, a small induced magnetic field is generated in the opposite direction, leading to a weak repulsion of the external field. This effect is very weak and generally masked by other magnetic effects if present.
Paramagnetism: Weak Attraction to Magnetic Fields
Paramagnetism occurs in atoms with unpaired electrons. These unpaired electrons have net magnetic moments that can align with an external magnetic field, resulting in a weak attraction. The alignment is random in the absence of an external field, but when a field is applied, the magnetic moments tend to align, creating a net magnetization. The strength of paramagnetism is directly proportional to the number of unpaired electrons. Paramagnetic materials lose their magnetization when the external field is removed.
Ferromagnetism: Strong Attraction and Permanent Magnetism
Ferromagnetism is a much stronger form of magnetism than paramagnetism. It's characterized by a strong attraction to magnetic fields and the ability to retain magnetization even after the external field is removed – the phenomenon of permanent magnetism. Ferromagnetism arises from a cooperative interaction between the magnetic moments of many atoms in a material. This interaction, called exchange interaction, favors parallel alignment of electron spins in neighboring atoms, leading to regions of spontaneous magnetization called magnetic domains.
Within each domain, the magnetic moments are aligned, creating a strong net magnetic moment. However, in an unmagnetized material, these domains are randomly oriented, canceling out each other's effect. When an external magnetic field is applied, the domains align with the field, resulting in a strong overall magnetization. This alignment persists even after the field is removed, giving rise to permanent magnetism. Examples of ferromagnetic materials include iron, nickel, and cobalt.
Other Types of Magnetism: Ferrimagnetism and Antiferromagnetism
Beyond the three primary types, there are other forms of magnetism:
-
Ferrimagnetism: Similar to ferromagnetism, ferrimagnetism involves parallel alignment of magnetic moments in domains. However, in ferrimagnetic materials, the magnetic moments of different atoms or ions are not equal in magnitude, resulting in a net magnetization, even though there's a degree of antiparallel alignment. Ferrites are a common example of ferrimagnetic materials.
-
Antiferromagnetism: In antiferromagnetic materials, the magnetic moments of neighboring atoms are aligned antiparallel, resulting in a net magnetization of zero. This occurs because the antiparallel alignment is energetically favorable due to exchange interactions. Antiferromagnetism is typically only observed at temperatures below a critical temperature called the Néel temperature.
Factors Affecting Atomic Magnetism: Temperature, Pressure, and Alloying
The magnetic properties of atoms and materials are not static; they are influenced by several factors:
Temperature
Temperature affects the degree of alignment of magnetic moments. Increasing temperature provides thermal energy that disrupts the alignment of magnetic moments, leading to a decrease in magnetization. In ferromagnetic materials, there exists a critical temperature called the Curie temperature. Above this temperature, the thermal energy overcomes the exchange interaction, and the material loses its ferromagnetic properties, becoming paramagnetic.
Pressure
Applying pressure can also influence the magnetic properties of a material. Pressure alters the interatomic distances and the strength of the exchange interactions, which can directly affect the magnetic order and transition temperatures.
Alloying
Alloying (mixing different metals) is a powerful technique for modifying magnetic properties. By carefully choosing the alloying elements, one can tune the magnetic properties, such as the Curie temperature, saturation magnetization, and coercivity (resistance to demagnetization).
Conclusion: A Complex Interplay of Quantum Effects
The magnetic properties of atoms are a consequence of the intricate interplay of electron spin and orbital angular momentum, governed by the laws of quantum mechanics. Understanding this interplay, including Hund's rules and the various types of magnetic interactions, is crucial for explaining the diverse magnetic behaviors observed in different materials. Furthermore, factors like temperature, pressure, and alloying can significantly modify these properties, offering opportunities for material design and technological applications. From the tiny magnetic moments of individual atoms to the powerful magnets we use daily, the story of atomic magnetism highlights the remarkable power of quantum mechanics in shaping the world around us.
Latest Posts
Related Post
Thank you for visiting our website which covers about What Causes Atoms To Have Magnetic Properties . We hope the information provided has been useful to you. Feel free to contact us if you have any questions or need further assistance. See you next time and don't miss to bookmark.