What Determines The Final Shape Of The Protein Molecule
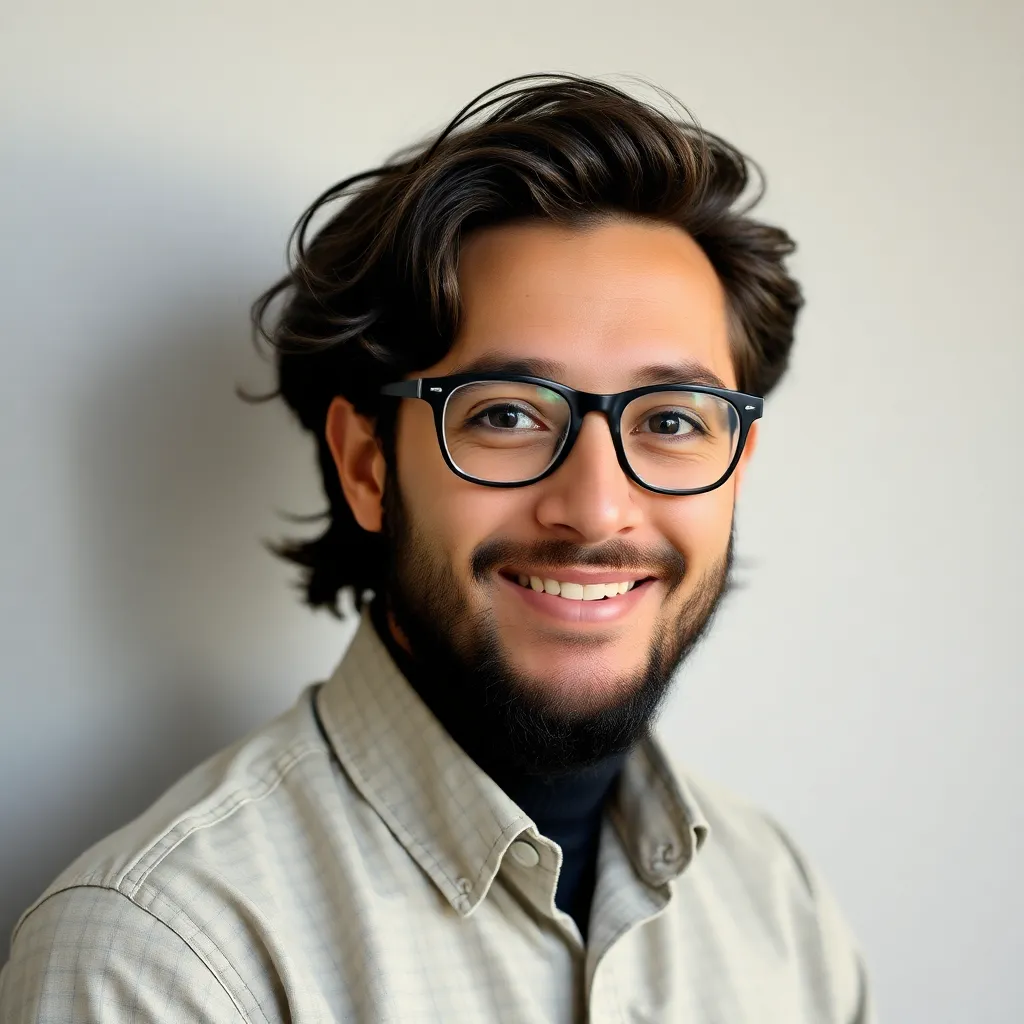
Muz Play
May 11, 2025 · 6 min read
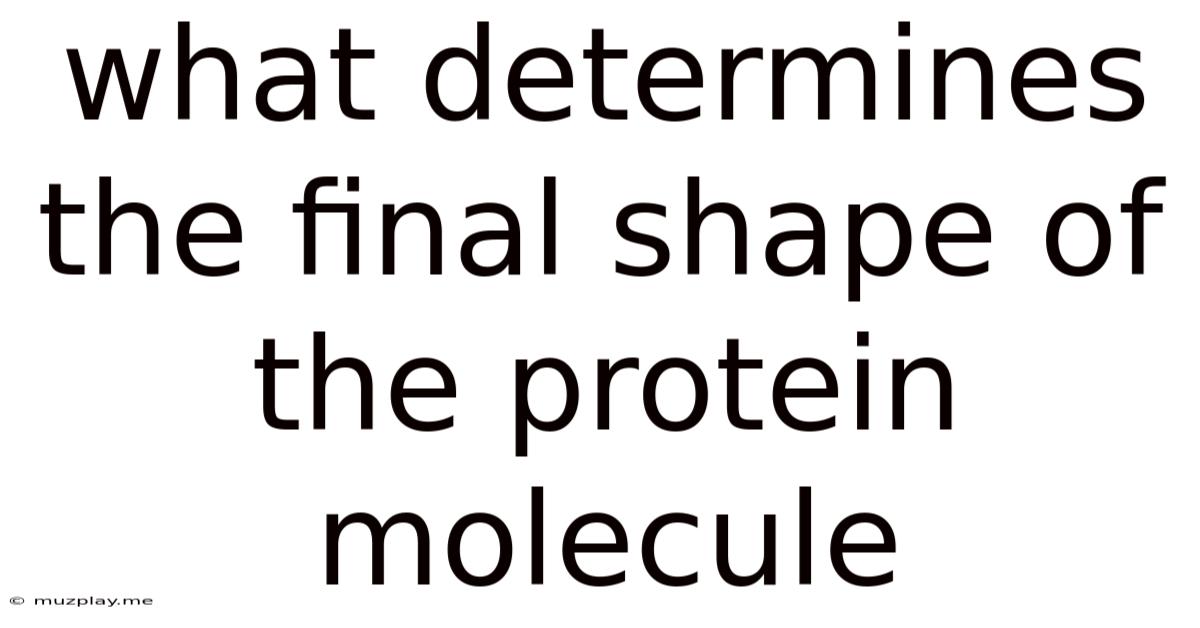
Table of Contents
What Determines the Final Shape of a Protein Molecule?
Proteins are the workhorses of the cell, carrying out a vast array of functions vital for life. From catalyzing biochemical reactions to providing structural support, the ability of a protein to perform its specific role is inextricably linked to its three-dimensional structure. But what precisely determines this intricate, final shape? The answer lies in a complex interplay of factors, starting at the level of the gene and extending to the protein's environment. This article will delve into the fascinating journey of a protein, from its genetic blueprint to its functional conformation, exploring the key determinants of its final shape.
The Primary Structure: The Foundation of Protein Shape
The journey begins with the primary structure, which is simply the linear sequence of amino acids. This sequence is dictated by the genetic code encoded in DNA. Each gene carries the instructions for synthesizing a specific polypeptide chain, a chain of amino acids linked together by peptide bonds. The order of these amino acids is crucial, as it determines all subsequent levels of protein structure. Even a single amino acid substitution can drastically alter the protein's final shape and function, as famously demonstrated in sickle cell anemia, where a single amino acid change in hemoglobin leads to a severe disease.
The Genetic Code and Amino Acid Sequence:
The genetic code is a system where codons (three-nucleotide sequences in mRNA) specify which amino acid is added to the growing polypeptide chain during translation. The sequence of codons in a gene, therefore, directly determines the amino acid sequence of the resulting protein. This sequence is not random; it's carefully orchestrated by the evolutionary process, honed over millions of years to produce proteins with precise shapes and functions.
Peptide Bonds and Polypeptide Chains:
The amino acids are linked together by peptide bonds, which are covalent bonds formed between the carboxyl group of one amino acid and the amino group of the next. This creates a long, unbranched polypeptide chain, the backbone of the protein. The R-groups (side chains) of the amino acids extend from this backbone, and their properties will significantly influence the protein's folding.
Secondary Structure: Local Folding Patterns
The primary structure doesn't reveal much about the protein's three-dimensional shape. The polypeptide chain begins to fold into local patterns called secondary structure, driven primarily by hydrogen bonds between the backbone atoms. Two common secondary structure elements are:
Alpha-Helices:
Alpha-helices are coiled structures stabilized by hydrogen bonds between the carbonyl oxygen of one amino acid and the amide hydrogen of the amino acid four residues down the chain. This creates a right-handed helix. The R-groups extend outwards from the helix, and their properties influence the helix's stability and overall contribution to the protein's shape. Certain amino acids, like proline, are helix breakers due to their rigid structure.
Beta-Sheets:
Beta-sheets are formed by hydrogen bonds between adjacent polypeptide strands, often running antiparallel (opposite directions). These strands are arranged side by side, creating a sheet-like structure. The R-groups alternate above and below the sheet plane, influencing its overall stability and interactions with other parts of the protein.
Loops and Turns:
Between the alpha-helices and beta-sheets are less structured regions called loops and turns. These regions are often flexible and play a crucial role in connecting secondary structure elements and determining the overall protein fold. They are often located on the protein's surface and involved in interactions with other molecules.
Tertiary Structure: The Three-Dimensional Arrangement
The tertiary structure represents the overall three-dimensional arrangement of a polypeptide chain, encompassing all secondary structure elements and their relative orientations. This level of structure is driven by a complex interplay of various forces:
Hydrophobic Interactions:
A major driving force in protein folding is the tendency of hydrophobic (water-fearing) amino acid side chains to cluster together in the protein's interior, away from the surrounding aqueous environment. This hydrophobic effect minimizes the contact of nonpolar residues with water, leading to a more thermodynamically stable conformation.
Hydrogen Bonds:
Hydrogen bonds between various parts of the polypeptide chain, including side chains and backbone atoms, contribute significantly to stabilizing the tertiary structure. These bonds are weaker than covalent bonds but collectively contribute to the overall stability of the folded protein.
Ionic Bonds (Salt Bridges):
Ionic bonds, or salt bridges, form between oppositely charged amino acid side chains. These interactions further stabilize the protein's three-dimensional structure by attracting negatively and positively charged groups.
Disulfide Bonds:
Disulfide bonds are covalent bonds formed between the sulfur atoms of cysteine residues. These strong bonds play a crucial role in stabilizing the tertiary structure, particularly in proteins secreted outside the cell, where they encounter oxidizing conditions.
Quaternary Structure: Multiple Polypeptide Chains
Some proteins consist of multiple polypeptide chains, each with its own tertiary structure. The arrangement of these individual subunits forms the quaternary structure. The interactions between subunits are similar to those involved in tertiary structure, involving hydrophobic interactions, hydrogen bonds, ionic bonds, and sometimes disulfide bonds. Examples of proteins with quaternary structure include hemoglobin (four subunits) and many enzymes.
Factors Influencing Protein Folding: Beyond the Sequence
While the amino acid sequence is the primary determinant of protein structure, other factors also play crucial roles:
Chaperones:
Molecular chaperones are proteins that assist in the proper folding of other proteins. They prevent aggregation and misfolding, guiding the nascent polypeptide chain towards its native conformation. Some chaperones, such as heat shock proteins, are upregulated under stress conditions to protect cells from protein denaturation.
Post-Translational Modifications:
After synthesis, proteins can undergo various post-translational modifications, such as glycosylation (addition of sugar moieties), phosphorylation (addition of phosphate groups), and ubiquitination (addition of ubiquitin molecules). These modifications can affect protein folding, stability, and function.
Environmental Factors:
The environment surrounding the protein also plays a role in its final shape. Factors like temperature, pH, and the presence of ions can influence protein folding and stability. Extreme changes in these conditions can lead to protein denaturation (unfolding), which can compromise its function.
Protein Misfolding and Disease
When proteins fail to fold correctly, they can misfold and aggregate, forming amyloid fibrils which are associated with a range of devastating diseases, including Alzheimer's disease, Parkinson's disease, and type II diabetes. These aggregates disrupt cellular function and can lead to cell death. Understanding the factors that influence protein folding is therefore crucial for developing strategies to prevent or treat these diseases.
Conclusion: A Complex and Dynamic Process
The determination of a protein's final shape is a remarkably intricate and dynamic process, starting with the genetic information encoded in DNA and extending to the protein's interactions with its environment. The interplay of primary, secondary, tertiary, and quaternary structures, guided by various forces and influenced by chaperones and environmental factors, results in the precisely folded protein that performs its specific biological function. Understanding this intricate process is fundamental to deciphering the mechanisms of life and developing strategies to address protein-related diseases. Further research into the intricacies of protein folding continues to expand our knowledge, offering new insights into the complexity and elegance of biological systems.
Latest Posts
Latest Posts
-
Donde Estaba Manuelita Cuando Murio Bolivar
May 12, 2025
-
Cellulose And Starch Are Examples Of What
May 12, 2025
-
Why Is Phenolphthalein Used As An Indicator
May 12, 2025
-
Como Sacar El Radio De Un Circulo
May 12, 2025
-
How Are Polymers Related To Monomers
May 12, 2025
Related Post
Thank you for visiting our website which covers about What Determines The Final Shape Of The Protein Molecule . We hope the information provided has been useful to you. Feel free to contact us if you have any questions or need further assistance. See you next time and don't miss to bookmark.