What Determines The Shape Of A Molecule
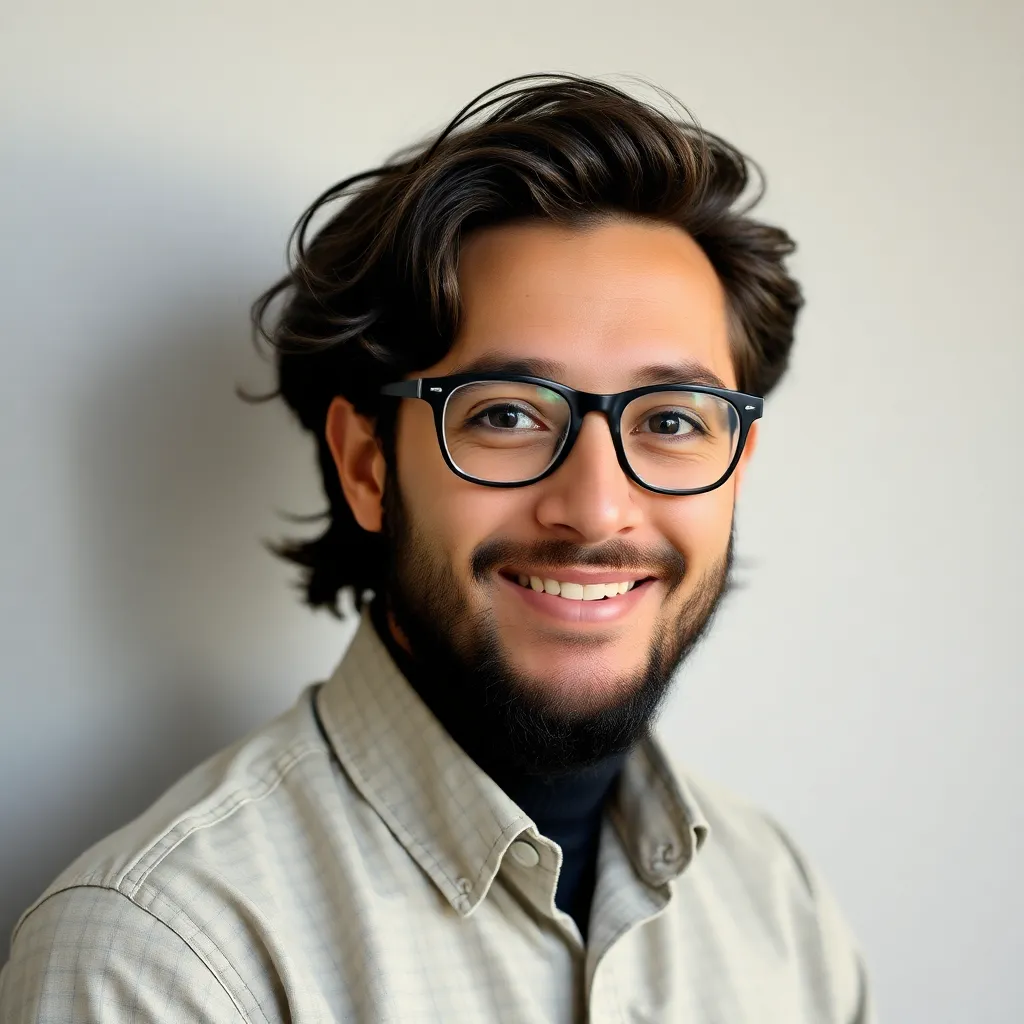
Muz Play
Mar 12, 2025 · 5 min read

Table of Contents
What Determines the Shape of a Molecule? A Deep Dive into Molecular Geometry
Understanding the shape of a molecule is fundamental to chemistry. It dictates a molecule's properties, influencing its reactivity, polarity, and even its physical state. But what exactly determines this crucial three-dimensional structure? The answer lies in a combination of factors, primarily the Valence Shell Electron Pair Repulsion (VSEPR) theory and the hybridization of atomic orbitals. This article will delve into these concepts, exploring how they interact to shape the molecules we encounter in the world around us.
The VSEPR Theory: Repulsion as the Guiding Principle
At the heart of molecular geometry lies the Valence Shell Electron Pair Repulsion (VSEPR) theory. This simple yet powerful theory posits that the shape of a molecule is determined by the repulsion between electron pairs in the valence shell of the central atom. These electron pairs, whether they are bonding pairs (shared between atoms) or lone pairs (not involved in bonding), strive to arrange themselves as far apart as possible to minimize repulsion. This optimal arrangement dictates the overall molecular geometry.
Predicting Molecular Shape with VSEPR
To predict the shape of a molecule using VSEPR, follow these steps:
-
Draw the Lewis structure: This shows the arrangement of atoms and valence electrons, identifying bonding and lone pairs.
-
Count the electron domains: An electron domain is a region of high electron density. This includes both bonding pairs and lone pairs around the central atom.
-
Determine the electron domain geometry: This describes the arrangement of electron domains around the central atom. Common electron domain geometries include linear (2 domains), trigonal planar (3 domains), tetrahedral (4 domains), trigonal bipyramidal (5 domains), and octahedral (6 domains).
-
Determine the molecular geometry: This describes the arrangement of atoms only, ignoring lone pairs. While the electron domain geometry influences the molecular geometry, lone pairs occupy more space than bonding pairs, leading to distortions in the molecular shape.
Examples:
-
Methane (CH₄): Carbon has four bonding pairs and no lone pairs. The electron domain geometry is tetrahedral, and the molecular geometry is also tetrahedral.
-
Ammonia (NH₃): Nitrogen has three bonding pairs and one lone pair. The electron domain geometry is tetrahedral, but the lone pair repels the bonding pairs more strongly, resulting in a trigonal pyramidal molecular geometry.
-
Water (H₂O): Oxygen has two bonding pairs and two lone pairs. The electron domain geometry is tetrahedral, but the two lone pairs cause significant repulsion, leading to a bent molecular geometry.
Hybridization: A Deeper Look into Atomic Orbitals
While VSEPR provides a good framework for predicting molecular shape, it doesn't fully explain the mechanism behind the arrangement of electron pairs. This is where hybridization comes in. Hybridization is the process of combining atomic orbitals within an atom to form new hybrid orbitals with different shapes and energies. These hybrid orbitals then participate in bonding.
Common Types of Hybridization
The type of hybridization depends on the number of electron domains around the central atom:
-
sp: Linear electron domain geometry (2 domains). Two hybrid orbitals are formed from one s and one p orbital. Examples include BeCl₂.
-
sp²: Trigonal planar electron domain geometry (3 domains). Three hybrid orbitals are formed from one s and two p orbitals. Examples include BF₃.
-
sp³: Tetrahedral electron domain geometry (4 domains). Four hybrid orbitals are formed from one s and three p orbitals. Examples include CH₄ and NH₃.
-
sp³d: Trigonal bipyramidal electron domain geometry (5 domains). Five hybrid orbitals are formed from one s, three p, and one d orbital. Examples include PCl₅.
-
sp³d²: Octahedral electron domain geometry (6 domains). Six hybrid orbitals are formed from one s, three p, and two d orbitals. Examples include SF₆.
The hybrid orbitals have specific orientations that directly correlate with the predicted molecular geometries from VSEPR theory. For example, the four sp³ hybrid orbitals in methane point towards the corners of a tetrahedron, explaining its tetrahedral shape.
Factors Beyond VSEPR and Hybridization
While VSEPR and hybridization are the primary determinants of molecular shape, other factors can also play a role:
-
Multiple Bonds: Double and triple bonds occupy more space than single bonds, leading to increased repulsion and potentially altering the molecular geometry slightly. For example, the bond angle in formaldehyde (H₂CO) is slightly less than 120° due to the double bond between carbon and oxygen.
-
Steric Effects: Bulky substituents can also influence molecular shape through steric hindrance. Large atoms or groups can repel each other, leading to distortions in bond angles and overall molecular conformation.
-
Intermolecular Forces: In some cases, intermolecular forces, such as hydrogen bonding, can influence the overall arrangement of molecules in a larger structure, affecting the macroscopic properties of a substance.
Advanced Concepts and Applications
The principles of VSEPR and hybridization are crucial for understanding a wide range of chemical phenomena:
-
Spectroscopy: Molecular shapes influence the vibrational and rotational modes of molecules, which are detected in spectroscopic techniques like infrared (IR) and Raman spectroscopy.
-
Reactivity: The shape of a molecule determines which sites are accessible for reactions, influencing the reaction mechanism and rate.
-
Polarity: The arrangement of atoms and bonds in a molecule dictates its overall polarity, affecting its solubility and other physical properties.
-
Biological Molecules: The intricate three-dimensional structures of proteins and nucleic acids, crucial for their biological functions, are determined by principles of molecular geometry and interactions between different parts of the molecules.
-
Materials Science: The shape of molecules significantly impacts the properties of materials. Designing molecules with specific shapes allows for the creation of materials with tailored properties.
Conclusion: A Holistic View of Molecular Shape
Determining the shape of a molecule is a complex process governed by several interacting factors. While VSEPR theory provides a simple yet effective framework for prediction, understanding hybridization offers a more mechanistic explanation. Furthermore, factors like multiple bonds, steric effects, and intermolecular forces can subtly influence the final molecular geometry. Mastering these concepts is fundamental for understanding chemical reactions, physical properties, and the behavior of molecules in diverse contexts, ranging from simple inorganic compounds to complex biological macromolecules and advanced materials. The interplay between VSEPR, hybridization, and other subtle influences paints a complete and fascinating picture of the world at the molecular level. Further exploration of these topics, particularly through practical exercises and visualization tools, will enhance your grasp of this fundamental aspect of chemistry.
Latest Posts
Latest Posts
-
Is Color An Intensive Or Extensive Property
May 09, 2025
-
Chemistry And Chemical Reactivity 10th Edition Pdf Free
May 09, 2025
-
What Was Significant About The New Habitats Darwin Visited
May 09, 2025
-
Are Melting Point And Freezing Point The Same
May 09, 2025
-
Do Protist Cells Have A Nucleus
May 09, 2025
Related Post
Thank you for visiting our website which covers about What Determines The Shape Of A Molecule . We hope the information provided has been useful to you. Feel free to contact us if you have any questions or need further assistance. See you next time and don't miss to bookmark.