What Does Quantized Mean In Chemistry
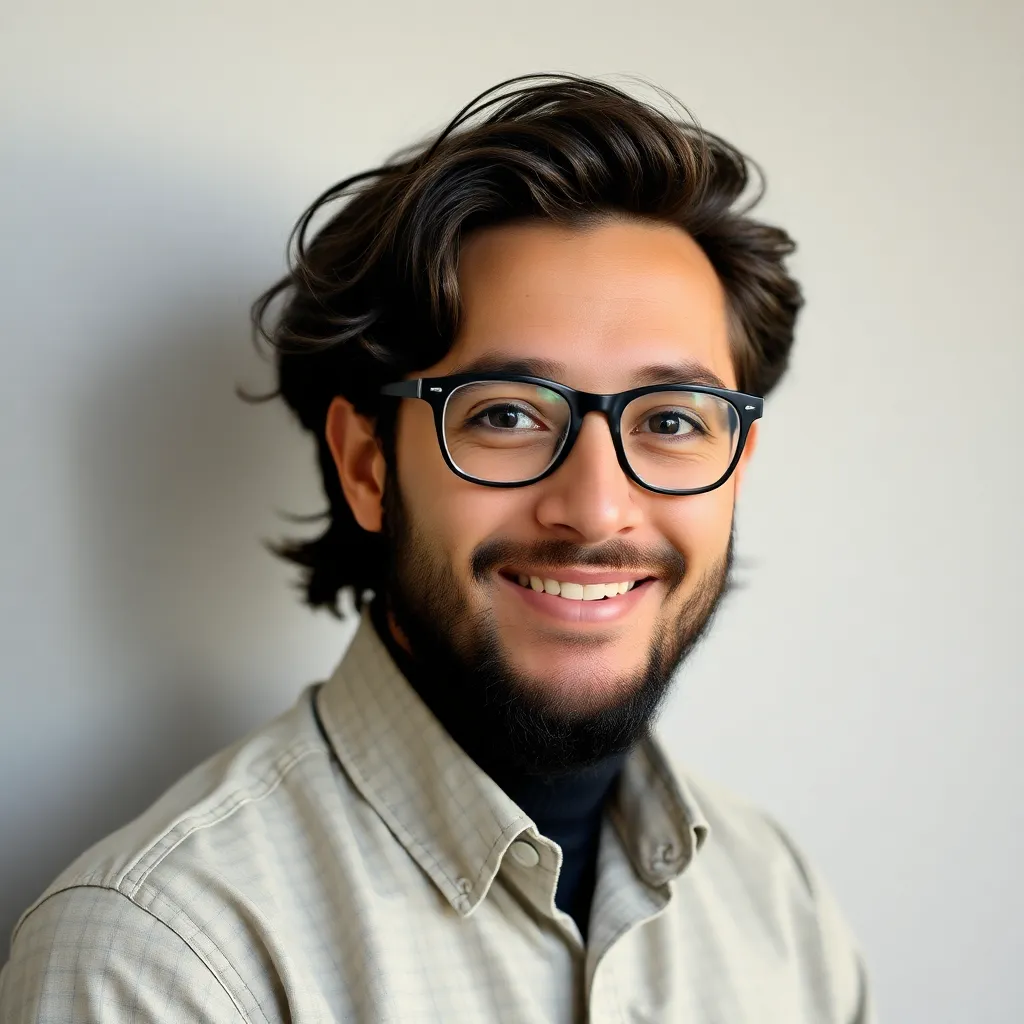
Muz Play
May 12, 2025 · 6 min read
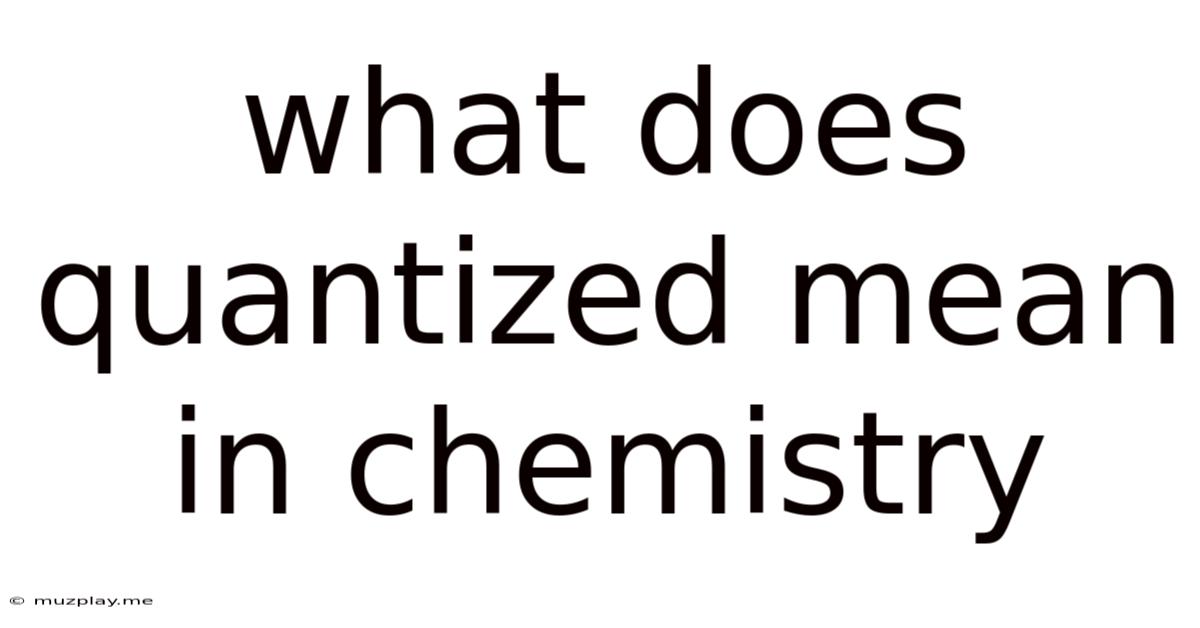
Table of Contents
What Does Quantized Mean in Chemistry? A Deep Dive into Quantum Phenomena
The word "quantized" might sound intimidating, particularly outside the realm of physics and chemistry. But understanding this fundamental concept is key to grasping the behavior of matter at the atomic and subatomic levels. This article will explore the meaning of "quantized" in chemistry, delving into its implications for various chemical processes and phenomena. We'll unpack the concept, exploring its historical context and demonstrating its relevance through numerous examples.
Understanding Quantization: A Departure from Classical Physics
In classical physics, energy, momentum, and other properties are considered continuous. This means they can take on any value within a given range. Imagine a ramp: you can stop at any point along its incline. This is not the case in the quantum world.
Quantization, in essence, means that certain properties, like energy, can only exist in discrete, specific amounts – much like steps on a staircase. You can't stand between steps; you're restricted to the available ones. These discrete amounts are called quanta.
This radical departure from classical physics was a cornerstone of the quantum revolution, beginning with Max Planck's explanation of blackbody radiation in 1900. Planck proposed that energy is not emitted or absorbed continuously but rather in discrete packets, or quanta, of energy, proportional to the frequency of the radiation. This revolutionary idea paved the way for a complete reimagining of our understanding of matter and energy.
The Birth of Quantum Theory: Planck's Constant
Central to the concept of quantization is Planck's constant (h), a fundamental physical constant approximately equal to 6.626 x 10<sup>-34</sup> joule-seconds. This constant dictates the size of the energy quanta. The smaller the value of h, the smaller the energy quanta, and the more closely the quantum world approximates the classical world. However, at the atomic and subatomic scales, h plays a crucial role, making quantization a dominant feature.
Quantization in Atomic Structure and Spectroscopy
The implications of quantization are profoundly evident in atomic structure and spectroscopy. Consider the Bohr model of the atom, a simplified but helpful illustration.
Electron Energy Levels: The Staircase Analogy
In the Bohr model, electrons orbit the nucleus only at specific, quantized energy levels. They can't exist in between these levels. This is analogous to our staircase: the electrons can only "stand" on specific steps (energy levels) and not in the spaces between them. When an electron transitions between energy levels, it absorbs or emits a photon of light whose energy precisely corresponds to the energy difference between the levels.
This explains the discrete nature of atomic spectra. When atoms are heated, their electrons jump to higher energy levels. As they fall back to lower levels, they emit light of specific frequencies (colors), creating a characteristic line spectrum. The discrete lines in the spectrum directly reflect the quantized energy levels of the electrons. This could not be explained using classical physics, which predicted a continuous spectrum.
The Quantum Mechanical Model: A More Accurate Description
The Bohr model, while helpful conceptually, is a simplification. The modern quantum mechanical model provides a more accurate, albeit mathematically complex, description of atomic structure. It uses the Schrödinger equation to calculate the probability of finding an electron at a particular location around the nucleus.
Even in this more sophisticated model, quantization remains crucial. Electrons are described by wave functions, which are solutions to the Schrödinger equation. Only certain wave functions are allowed, corresponding to specific energy levels and orbitals. This quantization of wave functions leads directly to the quantization of energy, momentum, and other properties of the electrons.
Quantization and Molecular Properties
The principles of quantization extend beyond individual atoms, significantly influencing molecular properties.
Molecular Vibrations and Rotations: Quantized Motions
Molecules, consisting of multiple atoms, possess quantized vibrational and rotational energy levels. These molecules vibrate and rotate at specific frequencies, determined by their structure and the forces between their atoms. Just like electrons in atoms, these vibrational and rotational energies are quantized, meaning they can only exist in discrete amounts.
The quantization of molecular vibrations and rotations is crucial for understanding molecular spectroscopy (infrared and microwave spectroscopy, respectively). The absorption or emission of photons by molecules during vibrational or rotational transitions produces discrete spectral lines, which provide information about the molecule's structure and dynamics.
Electronic Transitions in Molecules: Quantized Energy Changes
Similar to atoms, molecules also exhibit quantized electronic energy levels. Electrons in molecules can occupy different molecular orbitals, each associated with a specific energy. Transitions between these electronic energy levels, triggered by the absorption or emission of photons, result in the characteristic absorption and emission spectra of molecules (UV-Vis spectroscopy). The discrete nature of these spectra is a direct consequence of the quantization of electronic energy in molecules.
Quantization in Chemical Reactions and Catalysis
The implications of quantization extend to chemical reactions and catalysis.
Activation Energy and Reaction Rates: Quantized Barriers
Chemical reactions involve the breaking and formation of chemical bonds. The activation energy – the minimum energy required for a reaction to occur – is a quantized property. Reactant molecules must possess sufficient energy to overcome the activation energy barrier. Only molecules with energy equal to or greater than this quantized barrier can participate effectively in the reaction. This explains why reaction rates are often temperature-dependent: increasing temperature increases the number of molecules possessing the required activation energy.
Catalysis: Lowering Quantized Barriers
Catalysts accelerate chemical reactions by providing alternative reaction pathways with lower activation energies. Essentially, catalysts lower the quantized barrier that reactant molecules must overcome to proceed to products. By facilitating this process, catalysts enhance the reaction rate significantly.
Beyond the Basics: Advanced Implications of Quantization
The concept of quantization extends far beyond the introductory examples mentioned earlier.
Quantum Tunneling: A Non-Classical Phenomenon
Quantum tunneling is a remarkable phenomenon where a particle can pass through a potential energy barrier, even if it doesn't possess enough energy to overcome the barrier classically. This behavior, impossible in classical physics, arises directly from the wave-like nature of quantum particles. The probability of tunneling depends on the height and width of the barrier, and it demonstrates how quantization affects even seemingly insurmountable obstacles.
Quantum Superposition and Entanglement: Strange but Real
Quantum superposition refers to the ability of a quantum system to exist in multiple states simultaneously. An electron, for example, might be in a superposition of multiple orbitals until a measurement forces it into a definite state. This superposition principle is a direct consequence of the quantization of the electron's wave function.
Quantum entanglement is an even more mind-bending phenomenon where two or more quantum particles become linked, sharing the same fate regardless of the distance separating them. A measurement on one entangled particle instantly affects the state of the others, defying classical notions of locality. These concepts, while complex, underscore the profound implications of quantization in the quantum realm.
Conclusion: The Ubiquity of Quantization in Chemistry
In conclusion, "quantized" in chemistry signifies the discrete, specific nature of various properties at the atomic and molecular levels. This departure from classical physics has revolutionized our understanding of matter and energy, impacting numerous areas of chemistry. From atomic spectra to molecular vibrations, from reaction rates to catalytic processes, quantization plays a pivotal role in shaping chemical phenomena. The further exploration of quantum effects promises to unlock further advancements in various fields, from materials science and nanotechnology to medicine and computing. Understanding quantization is, therefore, not merely an academic exercise but a foundational requirement for anyone seeking a deeper understanding of the chemical world.
Latest Posts
Latest Posts
-
Which Non Nervous Tissue Develops From The Neuroectoderm
May 12, 2025
-
Charge Of Sodium Ion In Coulombs
May 12, 2025
-
Does Active Transport Go Against The Concentration Gradient
May 12, 2025
-
Characteristics Of Ecosystems With High Biodiversity
May 12, 2025
-
Is Membrane Bound Organelles Prokaryotic Or Eukaryotic
May 12, 2025
Related Post
Thank you for visiting our website which covers about What Does Quantized Mean In Chemistry . We hope the information provided has been useful to you. Feel free to contact us if you have any questions or need further assistance. See you next time and don't miss to bookmark.