What Does True Breeding Mean In Biology
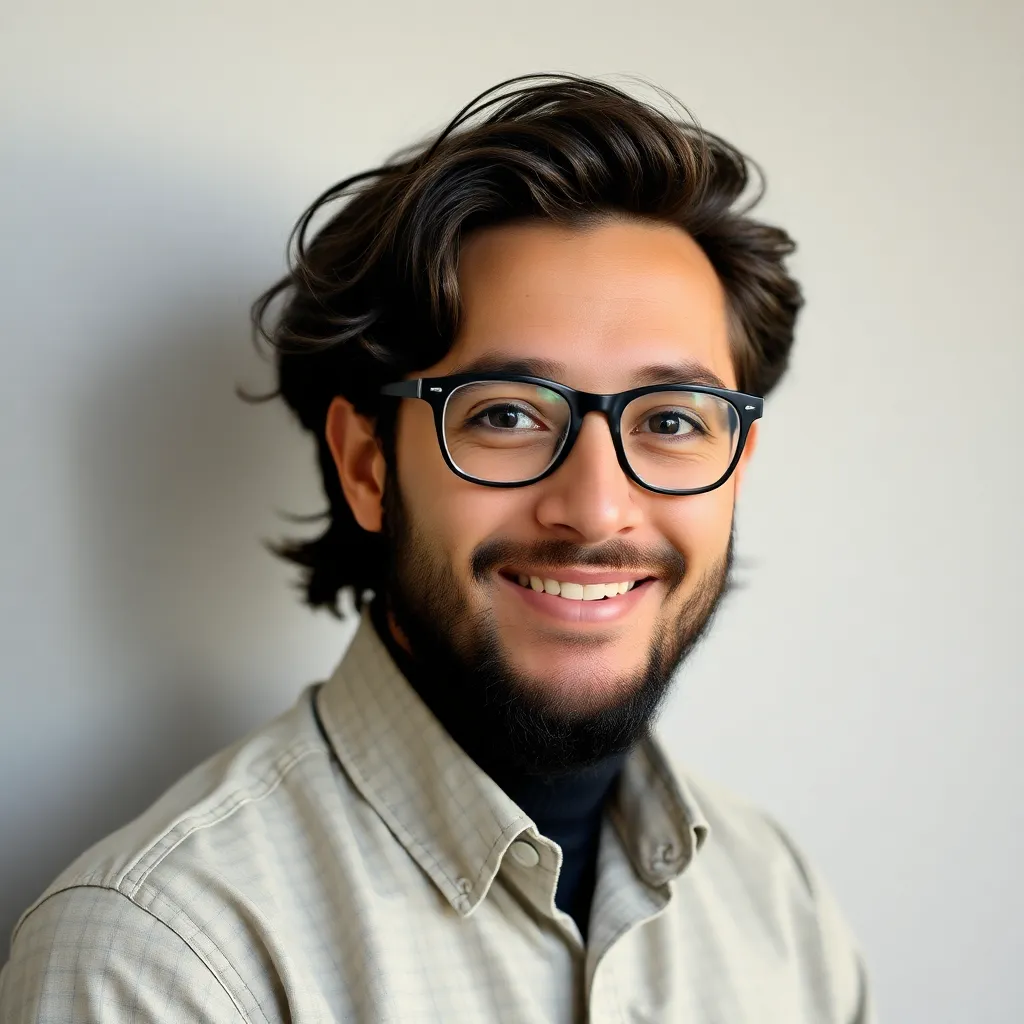
Muz Play
Apr 18, 2025 · 6 min read

Table of Contents
What Does True Breeding Mean in Biology? A Deep Dive into Homozygosity
True breeding. The term itself sounds almost mystical, conjuring images of perfectly predictable plant inheritance and unwavering genetic consistency. But what does it truly mean in the context of biology? Understanding true breeding is fundamental to grasping the principles of genetics, particularly Mendelian inheritance, and its implications extend far beyond the classroom, impacting fields like agriculture, biotechnology, and genetic research. This comprehensive article will delve into the definition of true breeding, exploring its significance, mechanisms, and practical applications.
Defining True Breeding: The Foundation of Genetic Predictability
In the simplest terms, true breeding refers to an organism that, when self-fertilized or crossed with another identical organism, consistently produces offspring with the same phenotype (observable characteristics) and genotype (genetic makeup) generation after generation. This unwavering consistency stems from the organism's homozygous nature—meaning it possesses two identical alleles for a particular gene. Alleles, remember, are variant forms of a gene that determine a specific trait.
Think of it like this: a true-breeding tall pea plant always produces tall offspring, not because it's magically tall, but because it carries two identical alleles for tallness (let's say 'TT'). When it self-pollinates or crosses with another 'TT' plant, all offspring inherit one 'T' allele from each parent, resulting in a uniform 'TT' genotype and consistently tall phenotype.
Conversely, a plant that's not true breeding for a trait might carry both a dominant (e.g., 'T' for tallness) and a recessive (e.g., 't' for shortness) allele ('Tt'). In this case, self-pollination or crossing with another 'Tt' plant leads to offspring displaying a mix of tall and short phenotypes, as per Mendelian inheritance patterns.
The Importance of Homozygosity
The cornerstone of true breeding is homozygosity. This genetic state ensures that the offspring inherit the same allele from both parents for the trait in question. The lack of genetic variation for that specific trait eliminates the possibility of unexpected phenotypic variations. This makes true breeding lines invaluable in genetic experiments and selective breeding programs.
Contrast with Heterozygosity: Heterozygous organisms, possessing two different alleles for a gene (e.g., 'Tt'), display a different inheritance pattern. Their offspring's phenotypes and genotypes are not predictable unless the dominant/recessive relationship between alleles is known.
The Mechanics of True Breeding: Self-Fertilization and Controlled Crosses
True breeding is typically achieved through two main methods: self-fertilization and controlled crosses.
1. Self-Fertilization: Nature's Own Breeding Program
Many plants, particularly those with flowers containing both male and female reproductive organs, can self-fertilize. This natural process allows a plant to reproduce without the need for external pollination. Through generations of self-fertilization, plants carrying homozygous alleles for specific traits become true breeding for those traits. This process naturally selects for homozygous combinations, effectively purifying the genetic line. Note, however, that self-fertilization can lead to inbreeding depression in some cases, reducing overall fitness due to the accumulation of harmful recessive alleles.
2. Controlled Crosses: The Human Hand in Genetic Purity
Scientists and breeders actively employ controlled crosses to establish true-breeding lines. This involves carefully selecting parent organisms with the desired homozygous trait and ensuring that they only reproduce with genetically identical individuals. This method allows for precise control over the genetic composition of the offspring and the rigorous selection of true breeding lines. This is especially important in agriculture, where creating true breeding varieties with desirable characteristics like high yield, disease resistance, and nutritional content is critical.
Applications of True Breeding: From Mendel's Peas to Modern Biotechnology
The concept of true breeding has far-reaching implications across various scientific disciplines and practical applications:
1. Mendelian Genetics: The Foundation of Inheritance Studies
Gregor Mendel's groundbreaking experiments relied heavily on true-breeding pea plants. By using these plants, he was able to establish fundamental principles of inheritance, including the concepts of dominant and recessive alleles, segregation, and independent assortment. The predictability of true-breeding lines allowed him to deduce the underlying genetic mechanisms driving trait inheritance, forming the foundation of modern genetics.
2. Plant Breeding: Cultivating Superior Crops
True breeding is a cornerstone of plant breeding. Breeders use controlled crosses and generations of selection to develop crop varieties with desirable traits such as high yield, disease resistance, improved nutritional content, and tolerance to harsh environmental conditions. True-breeding lines ensure that these traits are consistently passed down to subsequent generations, providing farmers with predictable and reliable crops.
3. Animal Breeding: Enhancing Livestock Traits
Similar to plant breeding, true breeding plays a crucial role in animal breeding. By carefully selecting and mating animals with desirable traits (e.g., milk production in dairy cows, meat yield in beef cattle), breeders can develop true-breeding lines with consistent and improved characteristics. This ensures the predictable inheritance of valuable traits and enhances the overall productivity and quality of livestock.
4. Genetic Research: Studying Gene Function and Interaction
True-breeding lines are indispensable tools in genetic research. The genetic uniformity of these lines minimizes variability, making it easier to study the effects of specific genes and their interactions with other genes and the environment. This simplified genetic background allows researchers to isolate and analyze the effects of individual genes more accurately.
5. Biotechnology: Developing Genetically Modified Organisms (GMOs)
The development of genetically modified organisms (GMOs) frequently utilizes true breeding lines as starting material. By modifying genes in a true-breeding line, researchers can introduce or modify traits with minimal genetic background noise. This allows them to assess the effects of specific gene alterations more effectively.
Challenges and Limitations of True Breeding
Despite its significant advantages, true breeding also faces certain challenges and limitations:
1. Inbreeding Depression: The Downside of Genetic Uniformity
The continuous self-fertilization or crossing of closely related individuals in true breeding can lead to inbreeding depression. This phenomenon occurs when harmful recessive alleles, previously masked in heterozygous individuals, become homozygous, resulting in reduced fitness, lower yields, and increased susceptibility to diseases. Breeders often employ strategies like outcrossing to mitigate the effects of inbreeding depression.
2. Reduced Genetic Diversity: A Vulnerability to Environmental Changes
True-breeding lines, by definition, exhibit limited genetic diversity. This lack of variation can make them vulnerable to environmental changes, pests, and diseases. A sudden shift in environmental conditions could severely impact a true-breeding population, leading to significant losses. Therefore, maintaining some degree of genetic diversity within breeding programs is crucial for long-term resilience.
3. Difficulty in Achieving True Breeding for Complex Traits: The Influence of Multiple Genes
While relatively easy to establish for single-gene traits, achieving true breeding for complex traits controlled by multiple genes is much more challenging. The interaction between multiple genes, as well as their interaction with environmental factors, makes the prediction of phenotype far more complex. Sophisticated statistical methods and advanced breeding techniques are often necessary to achieve true breeding for complex traits.
Conclusion: The Enduring Importance of True Breeding
True breeding remains a cornerstone of biology, particularly in the fields of genetics, agriculture, and biotechnology. Its significance lies in its ability to provide genetically uniform organisms with predictable phenotypes, which is crucial for research, breeding programs, and the development of new crop varieties and livestock breeds. While challenges associated with inbreeding depression and reduced genetic diversity exist, careful breeding practices and strategies can mitigate these risks, ensuring the continued relevance and importance of true breeding in advancing our understanding and application of genetic principles. The ability to create and utilize true-breeding lines showcases human ingenuity in manipulating the natural world to our benefit, highlighting the power of understanding fundamental biological principles and applying them to real-world problems.
Latest Posts
Latest Posts
-
Does The Entropy Of The Surroundings Increase For Spontaneous Processes
Apr 19, 2025
-
Construct An Mo Diagram For The He 2 Ion
Apr 19, 2025
-
Can The Zero Vector Be An Eigenvector
Apr 19, 2025
-
Concept Map Blood Groups And Transfusions
Apr 19, 2025
-
Which Set Of Compounds Illustrates The Law Of Multiple Proportions
Apr 19, 2025
Related Post
Thank you for visiting our website which covers about What Does True Breeding Mean In Biology . We hope the information provided has been useful to you. Feel free to contact us if you have any questions or need further assistance. See you next time and don't miss to bookmark.