What Gives Carbon The Ability To Form Chains
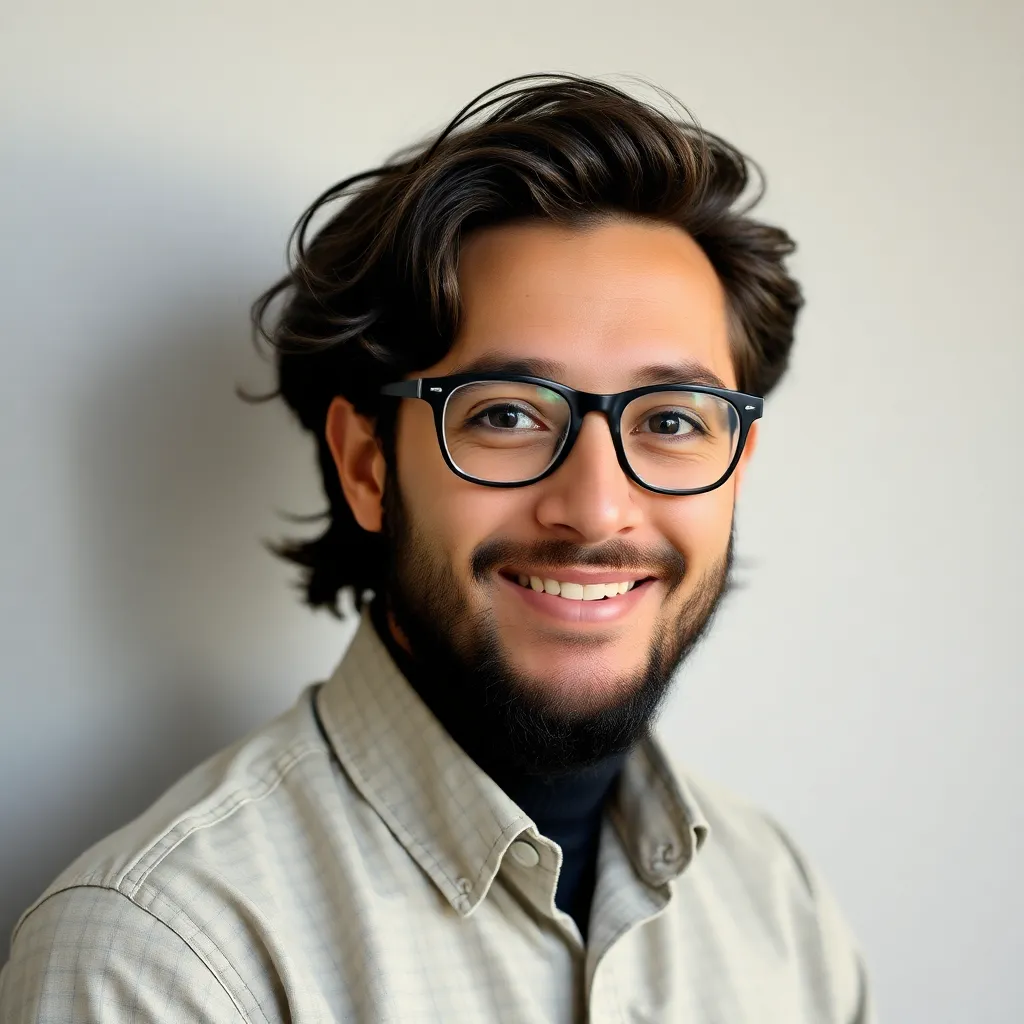
Muz Play
May 11, 2025 · 6 min read
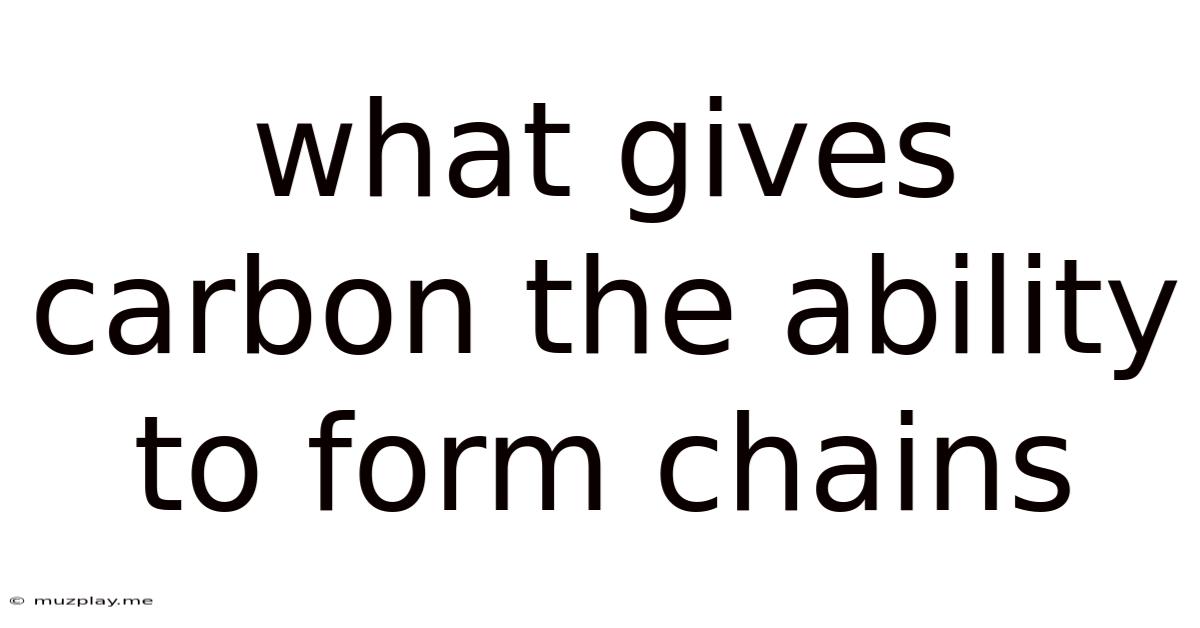
Table of Contents
What Gives Carbon the Ability to Form Chains? The Extraordinary Chemistry of Carbon
Carbon. The very word conjures images of diamonds, graphite, and the complex molecules of life. But what makes this element so unique, so versatile, that it forms the backbone of all known life and a vast array of other materials? The answer lies in its remarkable ability to form chains, a property stemming from its electronic structure and bonding capabilities. This article delves deep into the fascinating world of carbon's chemistry, exploring the reasons behind its chain-forming prowess and the implications of this ability for the diversity of organic and inorganic compounds.
The Electronic Structure: The Foundation of Carbon's Versatility
At the heart of carbon's chain-forming ability lies its electronic configuration. With an atomic number of 6, carbon possesses six electrons. Its electronic structure is 1s²2s²2p², meaning it has four electrons in its outer, or valence, shell. These four valence electrons are crucial because they participate in chemical bonding. The ability to form four bonds is fundamental to carbon's capacity to create extensive and diverse chains and networks.
The Significance of Four Valence Electrons
Unlike many other elements that achieve stability with fewer bonds, carbon readily forms four covalent bonds. A covalent bond involves the sharing of electron pairs between atoms. This sharing allows carbon to attain a stable octet configuration (eight electrons in its outer shell), mimicking the stable electron configuration of noble gases. This drive for stability is a key driving force behind carbon's reactivity and chain-forming capacity.
Hybridization: The Key to Carbon's Bonding Flexibility
To fully understand carbon's bonding versatility, we must consider the concept of hybridization. In simple terms, hybridization is the mixing of atomic orbitals to form new hybrid orbitals with different shapes and energies. This allows carbon to form different types of bonds, leading to various structural arrangements and ultimately, chain formation. The most common types of hybridization in carbon are:
-
sp³ Hybridization: In this hybridization, one s orbital and three p orbitals combine to form four sp³ hybrid orbitals. These orbitals are arranged tetrahedrally, pointing towards the corners of a tetrahedron. This arrangement is crucial for the formation of single bonds (sigma bonds) in alkanes, the simplest organic compounds. The tetrahedral geometry allows for the formation of long, flexible chains.
-
sp² Hybridization: Here, one s orbital and two p orbitals hybridize to form three sp² hybrid orbitals, leaving one p orbital unhybridized. These sp² orbitals are arranged in a trigonal planar geometry, with bond angles of approximately 120 degrees. This hybridization is common in alkenes, which contain carbon-carbon double bonds. The presence of a double bond restricts rotation around the carbon-carbon axis, influencing the overall shape and rigidity of the molecule.
-
sp Hybridization: In sp hybridization, one s orbital and one p orbital combine to form two sp hybrid orbitals, leaving two p orbitals unhybridized. The sp hybrid orbitals are arranged linearly, with a bond angle of 180 degrees. This hybridization is characteristic of alkynes, which possess carbon-carbon triple bonds. The linear geometry further impacts the molecule's structure and properties.
The ability of carbon to undergo different types of hybridization significantly enhances its capacity to form diverse chains and rings, contributing to the vastness and complexity of organic chemistry.
Carbon-Carbon Bond Strength: The Backbone of Chains
The strength of the carbon-carbon bond is another crucial factor facilitating chain formation. The carbon-carbon single bond (C-C) is relatively strong, allowing for the construction of long and stable carbon chains. This bond energy ensures that the chains do not readily break apart under normal conditions.
The Role of Covalent Bonding
The covalent nature of carbon-carbon bonds is pivotal. Electrons are shared between carbon atoms, resulting in a strong attraction that holds the atoms together. This sharing is more effective than other types of bonding, such as ionic bonding, in maintaining the integrity of the carbon chains.
Comparing Carbon-Carbon Bonds with Other Bonds
When compared to bonds between other elements, the carbon-carbon bond's strength and stability contribute to the unique chain-forming capacity of carbon. Many other elements form bonds that are weaker or more prone to breaking, limiting their ability to form long, stable chains.
The Diversity of Carbon Chains: Linear, Branched, and Cyclic
The ability of carbon to form four bonds allows for a remarkable variety of chain structures:
Linear Chains: The Simplest Structures
Linear chains consist of carbon atoms arranged in a straight line. These are the simplest type of carbon chain and form the basis of many organic molecules. Examples include the straight-chain alkanes like butane and pentane.
Branched Chains: Introducing Complexity
Branched chains involve carbon atoms branching off from the main chain. The introduction of branches adds complexity to the molecule's structure and properties. This branching significantly increases the number of possible isomers (molecules with the same chemical formula but different structures).
Cyclic Chains: Rings of Carbon Atoms
Cyclic chains, or rings, involve carbon atoms bonded together to form a closed loop. These rings can be three-membered (cyclopropane), four-membered (cyclobutane), and so on, each with unique properties. Cyclic structures are fundamental to many important biological molecules, such as sugars and some amino acids.
Beyond Chains: Carbon's Network Formation
While chain formation is a defining characteristic, carbon’s ability extends beyond linear and branched structures. It also forms extensive networks, contributing to materials with unique properties. Examples include:
Graphite: A Layered Network
Graphite, a form of carbon used in pencils, showcases carbon's network-forming ability. It consists of layers of carbon atoms arranged in hexagonal lattices. These layers are weakly bonded together, allowing them to slide over each other, giving graphite its lubricating properties.
Diamond: A Strong Three-Dimensional Network
Diamond, another allotrope of carbon, represents a three-dimensional network of carbon atoms bonded together tetrahedrally. This rigid, strong network accounts for diamond's exceptional hardness and high refractive index.
Fullerenes: Spherical and Tubular Structures
Fullerenes, such as buckminsterfullerene (C60), demonstrate carbon's capacity to form closed, spherical, or tubular structures. These unique structures have potential applications in various fields, including materials science and nanotechnology.
The Implications of Carbon's Chain-Forming Ability
The ability of carbon to form chains has profound implications across many areas:
The Basis of Organic Chemistry
The vast field of organic chemistry is essentially the study of carbon-containing compounds. Carbon's chain-forming ability is the foundation upon which this enormous field is built. Millions of organic compounds are known, each with its unique structure, properties, and functions.
The Foundation of Life
Life on Earth is carbon-based. The complex molecules of life, including proteins, carbohydrates, nucleic acids, and lipids, are all based on carbon chains. Carbon's versatility allows for the formation of the intricate structures necessary for the diverse functions within living organisms.
Materials Science and Nanotechnology
Carbon's chain-forming ability also plays a crucial role in materials science and nanotechnology. Scientists are exploring new carbon-based materials with unique properties for applications in electronics, energy storage, and other fields.
Conclusion: The Unique Chemistry of Carbon
The ability of carbon to form strong, stable chains is a consequence of its electronic structure, the strength of its bonds, and its ability to undergo various types of hybridization. This unique property is the driving force behind the extraordinary diversity of organic compounds, the foundation of life, and the development of advanced materials. The exploration of carbon's chemistry continues to reveal new possibilities, with ongoing research promising further advancements in various fields, solidifying carbon's position as the cornerstone element of chemistry and life itself.
Latest Posts
Latest Posts
-
Examples Of Rational Functions In Real Life
May 11, 2025
-
Gram Negative Bacteria Identification Flow Chart
May 11, 2025
-
What Type Of Bond Is Found In Carbohydrates
May 11, 2025
-
Is Pressure Directly Proportional To Temperature
May 11, 2025
-
Discuss The Medical Significance Of The Imvic Series Of Tests
May 11, 2025
Related Post
Thank you for visiting our website which covers about What Gives Carbon The Ability To Form Chains . We hope the information provided has been useful to you. Feel free to contact us if you have any questions or need further assistance. See you next time and don't miss to bookmark.