What Happens To Energy When The Wavelength Is Shortened
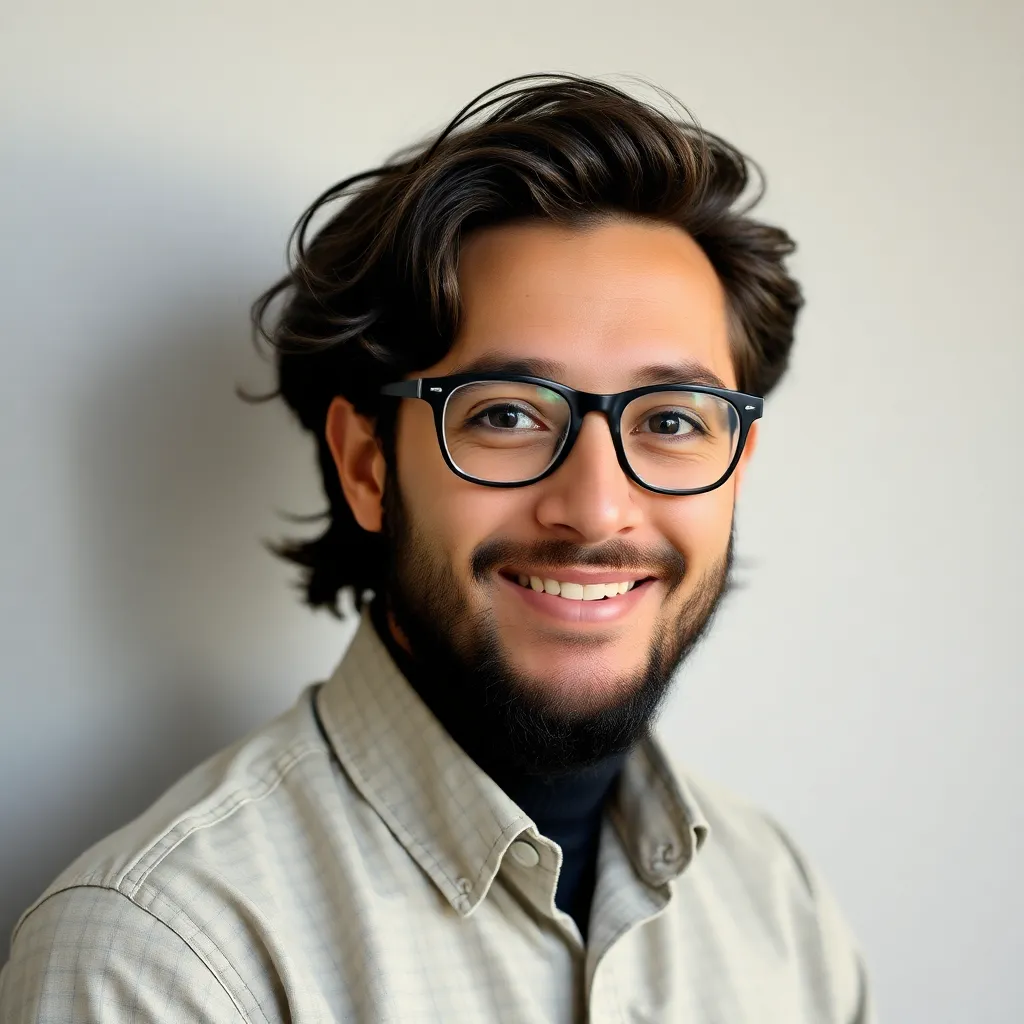
Muz Play
May 10, 2025 · 6 min read
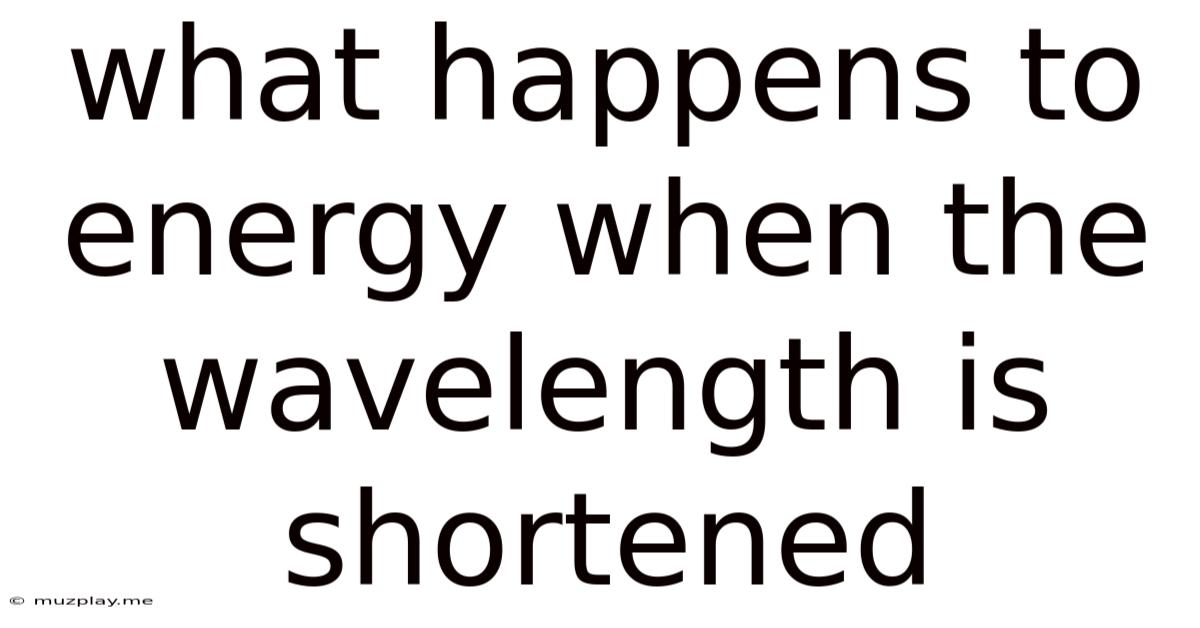
Table of Contents
What Happens to Energy When the Wavelength is Shortened?
The relationship between energy and wavelength is fundamental to our understanding of physics, particularly in the realms of light, electromagnetism, and quantum mechanics. This article delves into the intricacies of this relationship, exploring what happens to energy when the wavelength of electromagnetic radiation is shortened. We'll investigate the underlying physics, explore relevant examples, and discuss the implications across various scientific disciplines.
The Inverse Relationship: Energy and Wavelength
The cornerstone of understanding this phenomenon lies in the inverse relationship between energy (E) and wavelength (λ). This relationship is elegantly described by the equation:
E = hc/λ
where:
- E represents the energy of the photon (or quantum of electromagnetic radiation).
- h is Planck's constant (approximately 6.626 x 10^-34 Js), a fundamental constant in quantum mechanics.
- c is the speed of light in a vacuum (approximately 3 x 10^8 m/s).
- λ is the wavelength of the electromagnetic radiation.
This equation reveals that energy and wavelength are inversely proportional. As wavelength decreases (shortens), the energy of the radiation increases proportionally. Conversely, as wavelength increases, energy decreases. This seemingly simple equation has profound implications across numerous scientific fields.
Exploring the Electromagnetic Spectrum
The electromagnetic spectrum encompasses a broad range of wavelengths, from extremely long radio waves to incredibly short gamma rays. Each region of the spectrum corresponds to a specific range of energies. Let's examine how energy changes as we move across the spectrum towards shorter wavelengths:
Radio Waves: Low Energy, Long Wavelengths
Radio waves possess the longest wavelengths and, consequently, the lowest energies in the electromagnetic spectrum. They are used for communication technologies, broadcasting, and radar systems. Their low energy means they generally don't interact strongly with matter.
Microwaves: Slightly Higher Energy
Microwaves have shorter wavelengths than radio waves and therefore possess higher energy. This increased energy allows them to excite water molecules, which is the principle behind microwave ovens.
Infrared Radiation: Heating Effects
Infrared radiation has even shorter wavelengths and higher energy than microwaves. This is the type of radiation we feel as heat. Its energy is sufficient to cause vibrational and rotational transitions in molecules.
Visible Light: The Spectrum We See
Visible light, the small portion of the spectrum our eyes can detect, spans a relatively narrow range of wavelengths. Different wavelengths within this range correspond to different colors, with violet light having the shortest wavelengths (highest energy) and red light having the longest wavelengths (lowest energy).
Ultraviolet (UV) Radiation: Increased Energy, Potential Harm
Ultraviolet radiation possesses even shorter wavelengths and higher energy than visible light. This higher energy makes it capable of causing ionization and can be damaging to biological tissues, leading to sunburn and an increased risk of skin cancer. UV radiation is also used in sterilization techniques due to its ability to kill microorganisms.
X-rays: High Energy, Penetrating Power
X-rays have significantly shorter wavelengths and much higher energy than UV radiation. Their high energy allows them to penetrate soft tissues but are absorbed by denser materials like bone, making them valuable tools in medical imaging.
Gamma Rays: The Highest Energy
Gamma rays are at the extreme end of the spectrum, possessing the shortest wavelengths and highest energies. They are produced by radioactive decay and nuclear processes. Their high energy makes them extremely penetrating and potentially harmful to living organisms.
Implications Across Scientific Disciplines
The relationship between energy and wavelength has far-reaching implications across numerous scientific disciplines:
Astronomy and Astrophysics: Studying the Cosmos
Astronomers utilize the electromagnetic spectrum to study celestial objects. By analyzing the wavelengths of light emitted or absorbed by stars, galaxies, and other celestial bodies, scientists can determine their temperature, composition, and motion. The detection of high-energy radiation like X-rays and gamma rays reveals valuable information about energetic processes occurring in the universe, such as supernova explosions and active galactic nuclei.
Medicine: Diagnosis and Treatment
Medical applications heavily rely on the energy-wavelength relationship. X-rays provide valuable diagnostic tools, while gamma rays are used in radiotherapy to target and destroy cancerous cells. Laser technology, utilizing specific wavelengths of light, finds applications in various surgical procedures and therapies.
Materials Science: Studying Material Properties
The interaction of electromagnetic radiation with matter is influenced by the energy of the radiation. By studying how materials absorb, reflect, or transmit light of different wavelengths, scientists can determine the material's electronic structure, chemical composition, and other properties. This knowledge is crucial in designing new materials with specific optical properties.
Chemistry: Spectroscopy and Molecular Analysis
Spectroscopy, the study of the interaction of electromagnetic radiation with matter, relies heavily on the energy-wavelength relationship. By analyzing the absorption or emission spectra of molecules, chemists can identify the types of molecules present in a sample and determine their structure. Different wavelengths of light excite different vibrational and electronic transitions in molecules, providing detailed information about their properties.
Environmental Science: Remote Sensing and Pollution Monitoring
Remote sensing technologies utilize electromagnetic radiation to monitor environmental conditions. Satellites and aircraft carry sensors that measure the radiation reflected or emitted from the Earth's surface, providing information about vegetation, water quality, pollution levels, and other environmental parameters. Different wavelengths of radiation are sensitive to different features, allowing scientists to obtain comprehensive data.
Quantum Mechanical Interpretation
The equation E = hc/λ is not just an empirical observation; it arises directly from the quantum nature of light. Light, and all electromagnetic radiation, is composed of discrete packets of energy called photons. The energy of a photon is directly proportional to its frequency (ν) and inversely proportional to its wavelength:
E = hν = hc/λ
This equation highlights the quantized nature of energy. Only specific amounts of energy can be absorbed or emitted by atoms and molecules, corresponding to transitions between different energy levels. Shortening the wavelength corresponds to increasing the frequency and, consequently, increasing the energy of individual photons. This quantization is a fundamental concept in quantum mechanics and has profound implications for our understanding of the microscopic world.
Practical Applications and Examples
Several practical examples illustrate the consequences of shortening wavelengths and increasing energy:
- Sunburn: The higher energy of UV radiation compared to visible light explains why prolonged exposure to sunlight can lead to sunburn. The UV photons have enough energy to damage DNA in skin cells.
- Microwave ovens: Microwaves are used to heat food because their energy is sufficient to excite the water molecules in the food, causing them to vibrate and generate heat.
- Medical X-rays: The high energy of X-rays allows them to penetrate soft tissue, enabling doctors to obtain images of bones and internal organs.
- Laser cutting: Lasers use highly focused beams of light with specific wavelengths. Shortening the wavelength increases the energy, enhancing the laser's ability to cut through materials.
Conclusion
The inverse relationship between energy and wavelength of electromagnetic radiation is a fundamental principle with far-reaching consequences. As wavelength shortens, the energy of the radiation increases proportionally, impacting its interactions with matter and leading to a wide range of applications across various scientific disciplines. Understanding this relationship is crucial for advancing our knowledge of the universe, developing new technologies, and addressing critical challenges in fields such as medicine and environmental science. The quantum nature of light further underscores the profound implications of this simple yet powerful equation. Future research will continue to explore the intricate details of energy-wavelength interactions, opening up new possibilities for innovation and discovery.
Latest Posts
Latest Posts
-
A Noble Gas In Period 4
May 11, 2025
-
Waves Interact With And Other
May 11, 2025
-
Rotting Banana Chemical Or Physical Change
May 11, 2025
-
Atoms Combine In Whole Number Ratios
May 11, 2025
-
What Is A Proposition Of Policy
May 11, 2025
Related Post
Thank you for visiting our website which covers about What Happens To Energy When The Wavelength Is Shortened . We hope the information provided has been useful to you. Feel free to contact us if you have any questions or need further assistance. See you next time and don't miss to bookmark.