What Happens To The Electrons In Metallic Bonding
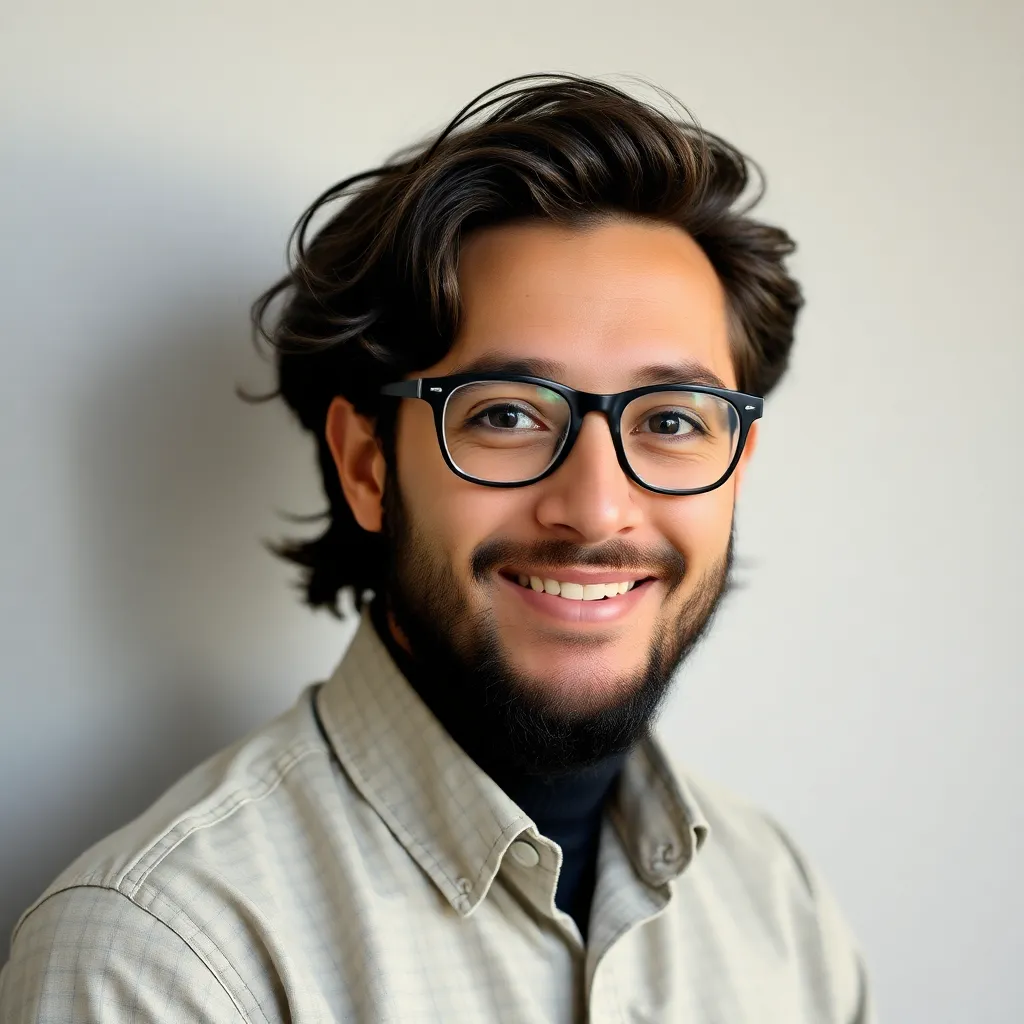
Muz Play
Apr 04, 2025 · 7 min read
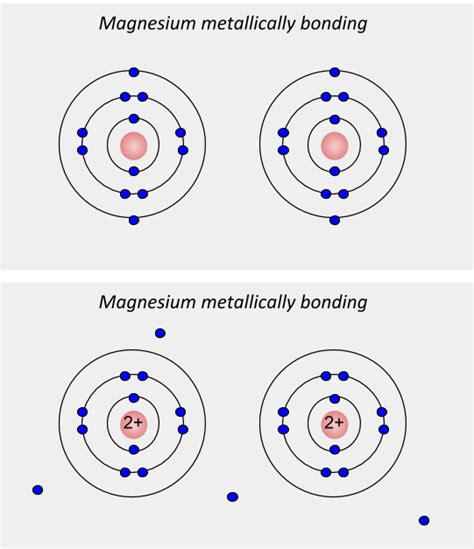
Table of Contents
What Happens to Electrons in Metallic Bonding? A Deep Dive
Metallic bonding is a unique type of chemical bonding that's responsible for many of the characteristic properties of metals, such as their excellent conductivity, malleability, and ductility. Unlike ionic or covalent bonding, where electrons are either transferred or shared between specific atoms, metallic bonding involves a sea of delocalized electrons. Understanding what happens to these electrons is key to understanding the behavior of metals.
The Sea of Electrons: A Delocalized Model
In a metallic solid, the valence electrons – the outermost electrons in an atom – are not associated with any particular atom. Instead, they are delocalized, meaning they are free to move throughout the entire metallic lattice. This creates a "sea" or "cloud" of electrons surrounding the positively charged metal ions. Imagine a lattice of positive ions submerged in a fluid of negatively charged electrons. This model is crucial for explaining the unique properties of metals.
How Delocalization Occurs
The delocalization of electrons arises from the relatively low ionization energies of metal atoms. These atoms readily lose their valence electrons, forming positively charged ions (cations). However, unlike in ionic bonding, these electrons are not transferred to another specific atom. Instead, they become shared among all the metal atoms in the structure. The attraction between these positively charged metal ions and the sea of delocalized electrons is what holds the metal together.
The quantum mechanical explanation lies in the overlapping of atomic orbitals. When metal atoms come together to form a solid, their valence atomic orbitals overlap extensively. This creates a large number of molecular orbitals that are spread throughout the entire crystal lattice. These molecular orbitals are very closely spaced in energy, forming essentially a continuous band of energy levels. The valence electrons occupy these delocalized molecular orbitals, allowing them to move freely throughout the metal.
The Implications of Delocalized Electrons: Explaining Metallic Properties
The delocalized nature of electrons in metallic bonding is directly responsible for several key properties exhibited by metals:
1. Electrical Conductivity
The ease with which metals conduct electricity is a direct consequence of the mobility of their delocalized electrons. When an electric field is applied across a metal, these electrons can readily move in the direction of the field, constituting an electric current. This is in stark contrast to ionic compounds, where the ions are fixed in their lattice positions and cannot move freely to carry a current. The higher the number of delocalized electrons, the better the conductivity of the metal.
2. Thermal Conductivity
Metals are also excellent conductors of heat. This is because the delocalized electrons can readily transfer kinetic energy throughout the metal. When one part of the metal is heated, the energized electrons collide with neighboring electrons and ions, rapidly distributing the heat energy throughout the material. This efficient heat transfer makes metals ideal for applications requiring rapid heat dissipation.
3. Malleability and Ductility
The ability of metals to be hammered into sheets (malleability) and drawn into wires (ductility) is another consequence of the sea of electrons. When a metallic solid is deformed, the metal ions can shift their positions relative to one another without disrupting the overall structure. This is because the delocalized electrons act as a "glue," holding the ions together even when their positions are altered. In contrast, ionic compounds are brittle because the disruption of the ionic lattice leads to strong electrostatic repulsions, causing fracture.
4. Luster (Metallic Shine)
The characteristic luster or shine of metals is also related to the interaction of light with the delocalized electrons. When light strikes a metal surface, the electrons absorb the light and then re-emit it. This re-emission of light gives metals their shiny appearance. The specific wavelength of light that's re-emitted determines the color of the metal. Many metals appear silvery because they re-emit light across a wide range of wavelengths.
5. High Melting and Boiling Points
The strong electrostatic attraction between the positively charged metal ions and the sea of delocalized electrons leads to relatively high melting and boiling points for most metals. A significant amount of energy is required to overcome this attraction and break the metallic bonds, resulting in the high temperatures needed to melt or boil the metal. However, the strength of the metallic bond varies depending on the number of delocalized electrons and the size of the metal ions. This variation explains the different melting and boiling points across various metals.
Factors Affecting Metallic Bonding Strength
The strength of metallic bonding is influenced by several factors:
-
Number of valence electrons: Metals with more valence electrons generally form stronger metallic bonds because there are more electrons contributing to the electron sea. For instance, transition metals with multiple valence electrons often have higher melting points than alkali metals with only one valence electron.
-
Size of the metal ions: Smaller metal ions lead to stronger metallic bonds because the electrostatic attraction between the ions and the delocalized electrons is stronger when the ions are closer together.
-
Charge density of the metal ions: A higher charge density (charge per unit volume) on the metal ions also strengthens the metallic bond.
Exceptions and Variations in Metallic Bonding
While the "sea of electrons" model provides a good overall picture of metallic bonding, it's important to acknowledge exceptions and variations. The model works best for simple metals like alkali and alkaline earth metals. However, transition metals and post-transition metals exhibit more complex bonding behaviors.
Transition Metals
Transition metals often have multiple valence electrons and partially filled d orbitals. This leads to stronger metallic bonds and contributes to their higher melting points and greater hardness compared to simple metals. The d electrons contribute to the electron sea, but they also participate in other bonding interactions, adding complexity to the bonding picture.
Alloys
Alloys are mixtures of two or more metals, and their properties can be significantly different from the constituent metals. The properties of alloys are influenced by the interactions between the different metal atoms and their electrons. Alloying can alter the strength, hardness, conductivity, and other properties of metals by changing the nature of the electron sea and the arrangements of the metal ions.
Semimetals (Metalloids)
Elements like silicon and germanium occupy a boundary between metals and non-metals. They exhibit some metallic characteristics but their bonding is not purely metallic. Their valence electrons are not completely delocalized, leading to lower electrical conductivity compared to true metals.
Applications of Understanding Metallic Bonding
The understanding of metallic bonding is fundamental to many scientific and technological applications:
-
Materials science: The design and development of new materials with specific properties, such as high strength alloys for aerospace applications or superconductors for electricity transmission.
-
Nanotechnology: The manipulation of materials at the nanoscale, where the properties of materials can be significantly different from their bulk counterparts, is strongly influenced by the understanding of metallic bonding at the atomic level.
-
Catalysis: Metallic catalysts play crucial roles in many chemical processes. The understanding of how the delocalized electrons interact with reactants is essential for designing efficient catalysts.
-
Corrosion engineering: Understanding metallic bonding helps in developing strategies to prevent corrosion, a major problem affecting metallic structures.
Conclusion: A Dynamic and Versatile Bonding Type
Metallic bonding is a fascinating and complex type of chemical bonding that is responsible for the unique properties of metals. The delocalized nature of electrons, forming a "sea" surrounding positively charged metal ions, is the key to understanding the electrical and thermal conductivity, malleability, ductility, and luster of metals. While the simple "sea of electrons" model provides a good starting point, the complexity of bonding increases with the inclusion of transition metals and the creation of alloys. Further research and a deeper understanding of metallic bonding are crucial for continued advancements in materials science, nanotechnology, and other fields. The study of this bonding type continues to reveal new insights and possibilities, making it a vibrant area of ongoing investigation.
Latest Posts
Latest Posts
-
Sex Linked Genes Are Located On
Apr 04, 2025
-
Which Organism Is An Animal Like Protist
Apr 04, 2025
-
Number Of Valence Electrons In Iron
Apr 04, 2025
-
Is The Normal Force Equal To The Weight
Apr 04, 2025
-
Which Target Organ Receives Dual Innervation
Apr 04, 2025
Related Post
Thank you for visiting our website which covers about What Happens To The Electrons In Metallic Bonding . We hope the information provided has been useful to you. Feel free to contact us if you have any questions or need further assistance. See you next time and don't miss to bookmark.