What Happens When A Solid Is Dissolved Into A Liquid
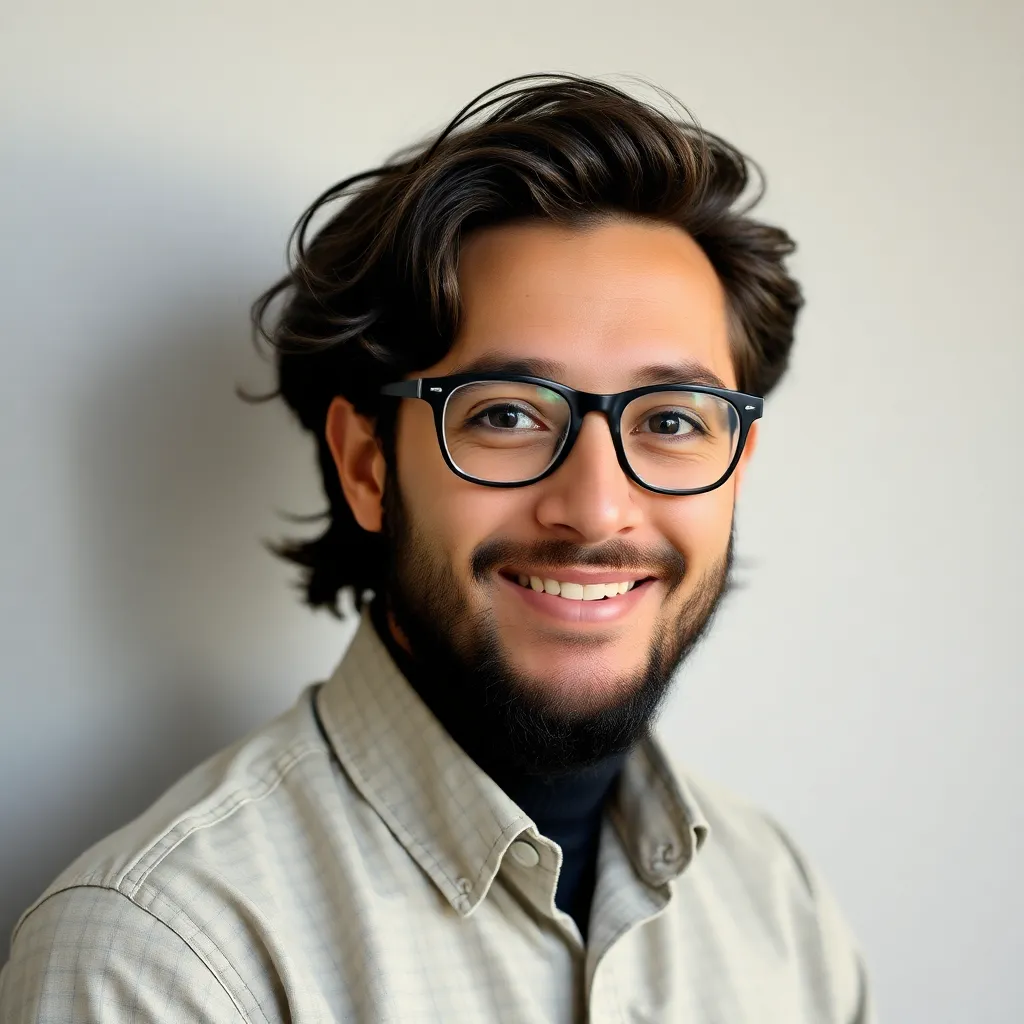
Muz Play
Apr 23, 2025 · 6 min read

Table of Contents
What Happens When a Solid is Dissolved into a Liquid? A Deep Dive into Dissolution
Dissolution, the process of a solid dissolving into a liquid, is a fundamental concept in chemistry with far-reaching implications in various fields, from medicine and environmental science to industrial processes and everyday life. Understanding this process requires delving into the intricate interplay of intermolecular forces, thermodynamics, and kinetics. This article will explore the complexities of dissolution, explaining the mechanisms involved and the factors influencing the rate and extent of the process.
The Molecular Dance: Intermolecular Forces and Dissolution
At the heart of dissolution lies the battle between intermolecular forces. When a solid is added to a liquid, the individual particles of the solid (typically ions or molecules) are held together by strong attractive forces, such as ionic bonds (in ionic compounds like salt) or hydrogen bonds (in many polar molecules like sugar). The liquid, on the other hand, also possesses intermolecular forces, like dipole-dipole interactions, London dispersion forces, or hydrogen bonds, depending on its nature.
For dissolution to occur, the attractive forces between the solute particles (the solid) must be overcome by the attractive forces between the solute particles and the solvent molecules (the liquid). This is often described as the solvation or hydration (if the solvent is water) process. Essentially, the solvent molecules surround the solute particles, isolating them from each other and pulling them into the liquid phase.
The Role of Polarity
The polarity of both the solute and the solvent plays a crucial role in determining whether dissolution will occur and how readily it happens. The famous adage "like dissolves like" encapsulates this principle. Polar solvents, like water, effectively dissolve polar solutes and ionic compounds due to their ability to form strong dipole-dipole interactions and ion-dipole interactions. The partial positive and negative charges on the water molecules interact strongly with the charged ions or polar molecules of the solute, leading to their separation and dispersion in the solution.
Nonpolar solvents, like hexane, on the other hand, tend to dissolve nonpolar solutes effectively. This is because the weak London dispersion forces between the nonpolar solvent and solute molecules are sufficient to overcome the weak intermolecular forces within the solute itself. Trying to dissolve a polar solute (like salt) in a nonpolar solvent (like oil) will usually result in little to no dissolution because the strong ion-ion interactions in the salt are not effectively overcome by the weak interactions with the nonpolar solvent molecules.
Thermodynamics of Dissolution: Energy Changes and Solubility
Dissolution is not simply a mechanical process; it's governed by thermodynamics. Two key factors determine whether a dissolution process will be spontaneous (occur naturally without external input): enthalpy change (ΔH) and entropy change (ΔS).
Enthalpy of Solution (ΔHsoln)
The enthalpy change of solution represents the heat absorbed or released during the dissolution process. It's the sum of three enthalpy changes:
-
Lattice energy (ΔHlattice): The energy required to break the bonds holding the solute particles together in the solid state. This is always positive (endothermic) as energy is required to overcome these attractive forces.
-
Enthalpy of solvation (ΔHsolvation): The energy released when the solvent molecules interact with the solute particles. This is usually negative (exothermic) as new attractive forces are formed.
-
ΔHsoln = ΔHlattice + ΔHsolvation
If the enthalpy of solvation is greater in magnitude than the lattice energy, the overall enthalpy change of solution is negative, indicating an exothermic process, where heat is released. Conversely, a positive ΔHsoln indicates an endothermic process, where heat is absorbed. While an exothermic process is favored, it's not the sole determinant of solubility.
Entropy of Solution (ΔSsoln)
Entropy, a measure of disorder, always increases during dissolution. The highly ordered arrangement of particles in the solid state is disrupted when the solute dissolves, leading to a more disordered, higher-entropy state in solution. This increase in entropy is a driving force for dissolution, as nature favors increased disorder.
Gibbs Free Energy and Solubility
The spontaneity of dissolution is ultimately determined by the Gibbs Free Energy change (ΔGsoln), which combines the effects of enthalpy and entropy:
ΔGsoln = ΔHsoln - TΔSsoln
Where T is the temperature in Kelvin. A negative ΔGsoln indicates a spontaneous process, meaning the solid will readily dissolve in the liquid. A positive ΔGsoln signifies a non-spontaneous process, meaning the solid will not dissolve significantly.
The solubility of a solid in a liquid is directly related to the Gibbs Free Energy of the dissolution process. A higher solubility corresponds to a more negative ΔGsoln.
Kinetics of Dissolution: Rate of Dissolution
While thermodynamics dictates whether dissolution will occur, kinetics determines how fast it happens. Several factors influence the rate of dissolution:
Surface Area
A larger surface area of the solid increases the contact between the solute and solvent, leading to faster dissolution. Crushing a solid into smaller particles significantly increases its surface area, thereby accelerating the process.
Stirring or Agitation
Stirring or agitation enhances the rate of dissolution by constantly bringing fresh solvent molecules into contact with the solid's surface. This replaces the solvent molecules that have already interacted with the solute, preventing the formation of a saturated layer around the solid.
Temperature
Increasing the temperature usually accelerates the rate of dissolution. Higher temperatures provide solvent molecules with more kinetic energy, leading to more frequent and energetic collisions with the solute particles. This facilitates the breaking of solute-solute interactions and the formation of solvent-solute interactions. However, the effect of temperature on solubility is complex and depends on whether the dissolution process is exothermic or endothermic.
Particle Size
Smaller particles dissolve faster than larger particles. This is because smaller particles have a larger surface area to volume ratio, allowing for more contact points between the solvent and solute.
Factors Affecting Solubility: Pressure and Other Considerations
Besides temperature, pressure also plays a role in solubility, although its effect is usually minor for solids dissolving in liquids. However, for gases dissolving in liquids, pressure has a significant impact, as described by Henry's Law.
Other factors also subtly influence solubility:
-
Presence of other solutes: The presence of other dissolved substances can either enhance or hinder the solubility of a given solid. Common-ion effect, for instance, reduces the solubility of a sparingly soluble salt.
-
pH: The pH of the solution can significantly affect the solubility of certain compounds, particularly those that are weak acids or bases.
-
Complexation: The formation of complexes between the solute and other molecules in the solution can alter solubility.
Applications of Dissolution: Real-World Examples
The principles of dissolution are fundamental to countless processes in various fields:
-
Pharmaceuticals: Dissolution is crucial for drug delivery. The rate at which a drug dissolves determines its bioavailability—how effectively the body can absorb and utilize it.
-
Environmental science: The dissolution of pollutants in water bodies is vital for understanding their environmental impact and designing remediation strategies.
-
Geochemistry: The dissolution and precipitation of minerals are essential processes shaping the Earth's crust.
-
Industrial processes: Many industrial processes, such as the production of chemicals and materials, rely on the controlled dissolution of solids in liquids.
-
Food science: The dissolution of various compounds in food contributes to its taste, texture, and nutritional value.
Conclusion: A Complex and Essential Process
Dissolution is a multifaceted process governed by a complex interplay of intermolecular forces, thermodynamic principles, and kinetic factors. Understanding these interactions is crucial for controlling and optimizing dissolution processes across a wide range of applications. From developing efficient drug delivery systems to managing environmental pollution, the principles discussed here offer a foundational understanding of this vital process. Further research continues to refine our understanding of dissolution, particularly concerning the complex behavior of solutes in diverse solvent systems and under various conditions.
Latest Posts
Latest Posts
-
Graph The Complex Conjugate Of The Given Number
Apr 23, 2025
-
Trust Thyself Every Heart Vibrates To That Iron String Meaning
Apr 23, 2025
-
Compare And Contrast Intermolecular Forces And Describe Intramolecular Forces
Apr 23, 2025
-
Photosystem I And Photosystem Ii Are Part Of
Apr 23, 2025
-
How Many Valence Electrons Are In Group 15
Apr 23, 2025
Related Post
Thank you for visiting our website which covers about What Happens When A Solid Is Dissolved Into A Liquid . We hope the information provided has been useful to you. Feel free to contact us if you have any questions or need further assistance. See you next time and don't miss to bookmark.