What Happens When An Electrical Impulse Reaches A Neuron's Ending
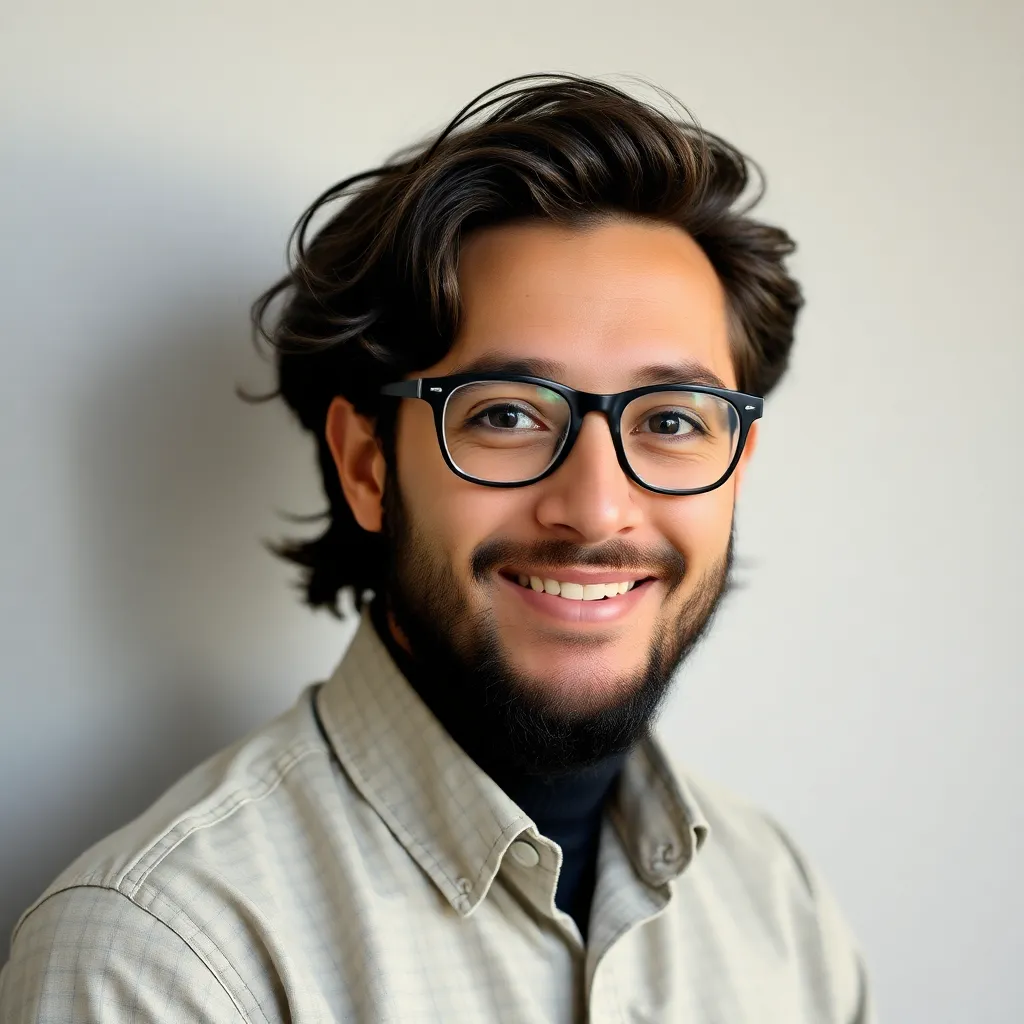
Muz Play
May 10, 2025 · 6 min read
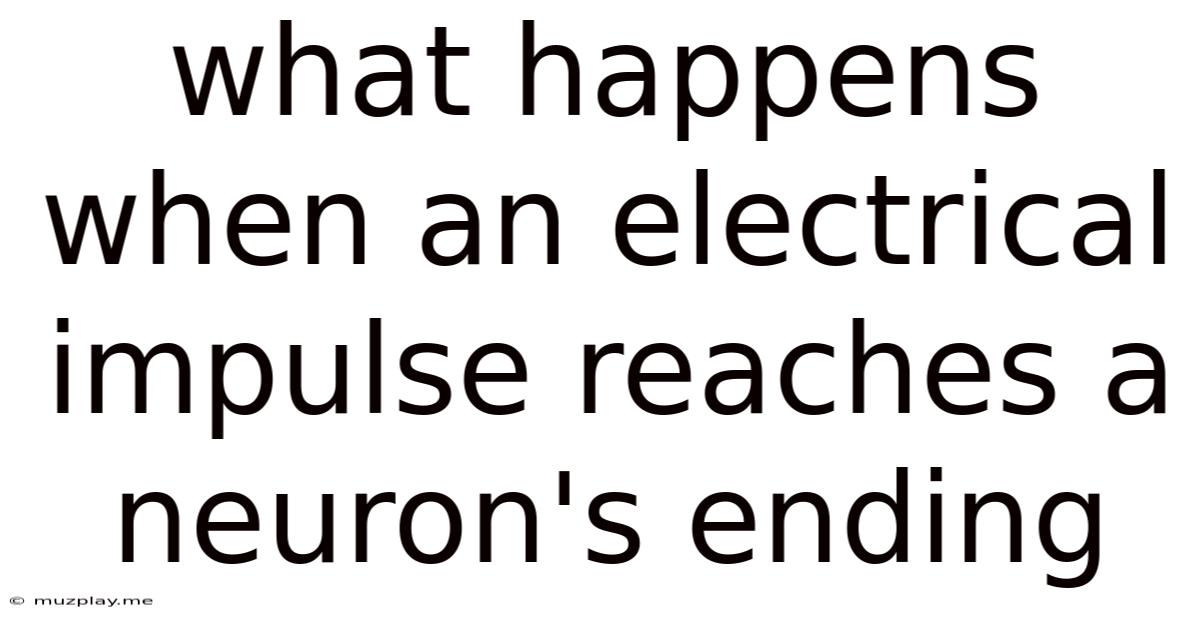
Table of Contents
What Happens When an Electrical Impulse Reaches a Neuron's Ending?
The human nervous system, a marvel of biological engineering, relies on the precise transmission of information between billions of neurons. This communication doesn't happen through direct physical contact, but rather through a sophisticated process involving electrical impulses and chemical messengers. Understanding what happens when an electrical impulse, or action potential, reaches a neuron's ending – the axon terminal – is crucial to grasping the intricacies of neural function and its implications for everything from thought and movement to sensation and emotion. This article delves deep into this fascinating process, exploring the intricate steps involved in neurotransmission and its potential malfunctions.
The Arrival of the Action Potential: A Cascade of Events
The journey of an action potential begins at the neuron's cell body (soma), where it's initiated by a stimulus exceeding the neuron's threshold potential. This electrical signal, a wave of depolarization, swiftly travels down the axon, the neuron's long, slender projection. The speed of this transmission is significantly influenced by factors like axon diameter (larger diameter = faster transmission) and the presence of myelin sheaths (insulating layers that facilitate saltatory conduction – jumping between Nodes of Ranvier).
Once the action potential reaches the axon terminal, a specialized region at the neuron's end, a series of events unfolds, leading to communication with the next neuron or target cell. This communication, called synaptic transmission, is not electrical but chemical, relying on the release of neurotransmitters.
1. Depolarization of the Axon Terminal: Opening Voltage-Gated Calcium Channels
Upon arrival of the action potential, the axon terminal's membrane potential undergoes depolarization. This change in voltage critically triggers the opening of voltage-gated calcium channels. These channels, embedded in the axon terminal membrane, are selectively permeable to calcium ions (Ca²⁺). The concentration of Ca²⁺ is significantly higher outside the neuron than inside. Therefore, when these channels open, calcium ions rush into the axon terminal, driven by the electrochemical gradient. This influx of Ca²⁺ is the pivotal event that initiates neurotransmitter release.
2. Vesicle Fusion and Neurotransmitter Release: The Role of SNARE Proteins
The axon terminal contains numerous synaptic vesicles, small membrane-bound sacs filled with neurotransmitters. These neurotransmitters are the chemical messengers that will carry the signal across the synaptic cleft, the gap between the presynaptic neuron (the neuron sending the signal) and the postsynaptic neuron (the neuron receiving the signal).
The influx of Ca²⁺ triggers a cascade of intracellular events culminating in the fusion of synaptic vesicles with the presynaptic membrane. This fusion process is orchestrated by a complex interplay of proteins, primarily SNARE proteins. These proteins act as molecular "docking machinery," precisely aligning the vesicle membrane with the presynaptic membrane. Once aligned, the vesicle membrane fuses with the presynaptic membrane, releasing the neurotransmitters into the synaptic cleft via exocytosis.
The amount of neurotransmitter released is directly proportional to the amount of Ca²⁺ entering the axon terminal. A stronger action potential, leading to a greater influx of Ca²⁺, results in the release of more neurotransmitters. This mechanism allows the nervous system to finely tune the strength of its signals.
3. Diffusion Across the Synaptic Cleft: Reaching the Postsynaptic Neuron
Once released into the synaptic cleft, the neurotransmitters diffuse across this narrow gap towards the postsynaptic neuron. The synaptic cleft is typically only about 20-40 nanometers wide, ensuring efficient diffusion. The concentration of neurotransmitters and the diffusion rate are crucial factors in determining the speed and strength of signal transmission.
4. Binding to Postsynaptic Receptors: Initiating a Response
The postsynaptic membrane of the receiving neuron contains numerous receptors specifically designed to bind to the released neurotransmitters. These neurotransmitter receptors are typically transmembrane proteins that undergo conformational changes upon neurotransmitter binding. This binding event initiates a series of events within the postsynaptic neuron.
The nature of the postsynaptic response depends on the type of receptor and the neurotransmitter involved. Some receptors are ionotropic, meaning they directly open or close ion channels upon binding, causing a rapid change in the postsynaptic membrane potential (either excitatory or inhibitory). Others are metabotropic, triggering a slower, but often more prolonged, response via intracellular signaling pathways involving second messengers.
5. Signal Termination: Ensuring Precise Communication
To ensure the precise and timely transmission of signals, the action of neurotransmitters must be terminated. This is achieved through several mechanisms:
- Reuptake: Neurotransmitters are actively transported back into the presynaptic neuron via transporter proteins. This reuptake allows the neuron to recycle the neurotransmitters.
- Enzymatic degradation: Enzymes in the synaptic cleft break down the neurotransmitters into inactive metabolites. For example, acetylcholinesterase breaks down acetylcholine.
- Diffusion: Some neurotransmitters simply diffuse away from the synapse, becoming diluted and less effective.
These termination mechanisms prevent prolonged stimulation of the postsynaptic neuron, ensuring the system remains responsive and capable of processing new signals.
Types of Synapses and Their Functional Significance
The process described above primarily focuses on chemical synapses, which are the most prevalent type of synapse in the nervous system. However, it's important to acknowledge the existence of electrical synapses, which allow for direct electrical coupling between neurons.
In electrical synapses, the pre- and postsynaptic neurons are connected via gap junctions, specialized channels that allow ions to flow directly between the cells. This type of synapse enables rapid, bidirectional transmission of signals, making it ideal for situations requiring fast, synchronized activity, such as escape reflexes.
The Implications of Dysfunction: Neurological and Psychiatric Disorders
Proper functioning of synaptic transmission is paramount for health. Disruptions in any stage of this process can lead to a range of neurological and psychiatric disorders.
-
Neurotransmitter imbalances: Imbalances in neurotransmitter levels or receptor function are implicated in conditions such as depression, anxiety, schizophrenia, and Parkinson's disease. Many medications used to treat these disorders aim to modulate neurotransmitter levels or receptor activity.
-
Autoimmune diseases: Autoimmune diseases can target components of the synapse, leading to impaired synaptic transmission.
-
Neurodegenerative diseases: Conditions like Alzheimer's disease and Huntington's disease are characterized by progressive neuronal loss and synaptic dysfunction.
-
Stroke: Stroke can damage neurons and disrupt synaptic transmission, leading to neurological deficits.
-
Drug addiction: Many addictive drugs exert their effects by altering synaptic transmission, often by affecting neurotransmitter release, reuptake, or receptor binding.
Further Research and Future Directions
The study of synaptic transmission is a vibrant and evolving field. Ongoing research continues to uncover new details about the molecular mechanisms involved, the diversity of neurotransmitters and receptors, and the complex interplay between different neuronal circuits. Advances in imaging techniques, genetic engineering, and computational modeling are providing increasingly sophisticated tools to explore this critical process.
Understanding the intricacies of synaptic transmission is not only essential for comprehending the normal function of the nervous system, but it's also crucial for developing effective treatments for a wide range of neurological and psychiatric disorders. Future research promises to further illuminate this fundamental aspect of brain function and pave the way for innovative therapeutic strategies.
Conclusion: A Symphony of Molecular Events
The arrival of an electrical impulse at a neuron's ending initiates a precisely orchestrated cascade of molecular events, leading to the release of neurotransmitters and communication with the next cell. This process, meticulously refined over millennia of evolution, is fundamental to every aspect of our nervous system's function. From simple reflexes to complex cognitive processes, it's the foundation upon which our thoughts, feelings, and actions are built. Further exploration of this remarkable process promises to unlock deeper insights into the complexities of the brain and provide new avenues for treating neurological and psychiatric conditions. The ongoing research in this field is vital for advancing our understanding of the human brain and improving human health.
Latest Posts
Latest Posts
-
Can Kinetic Energy Be Changed Without A Change In Temperature
May 10, 2025
-
If Oxygen Is Present Metabolism Is Considered To Be
May 10, 2025
-
Which Mineral Is The Most Abundant In The Body
May 10, 2025
-
What Happens When Oceanic And Continental Crust Collide
May 10, 2025
-
At What Type Of Boundary Do Rift Basins Form
May 10, 2025
Related Post
Thank you for visiting our website which covers about What Happens When An Electrical Impulse Reaches A Neuron's Ending . We hope the information provided has been useful to you. Feel free to contact us if you have any questions or need further assistance. See you next time and don't miss to bookmark.