What Happens When The Phosphate Bonds Of Atp Break
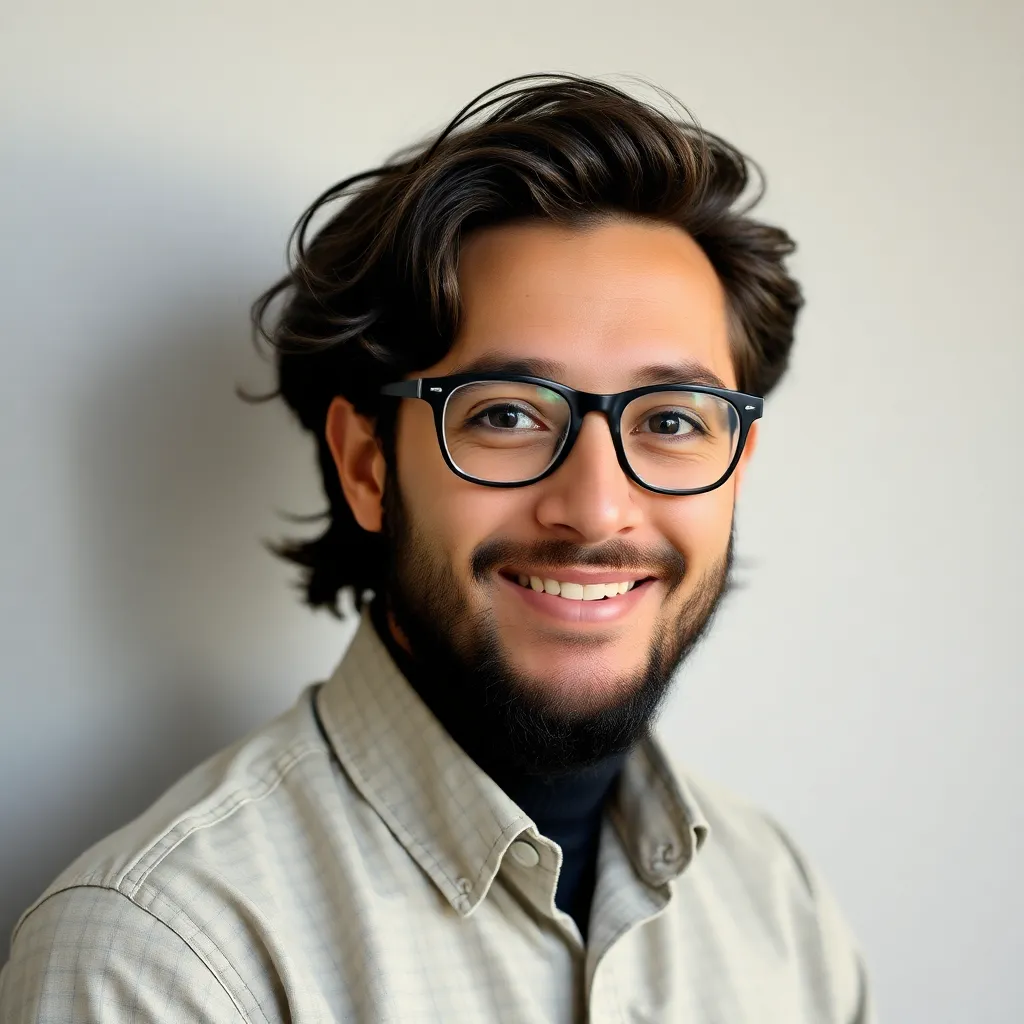
Muz Play
May 12, 2025 · 5 min read
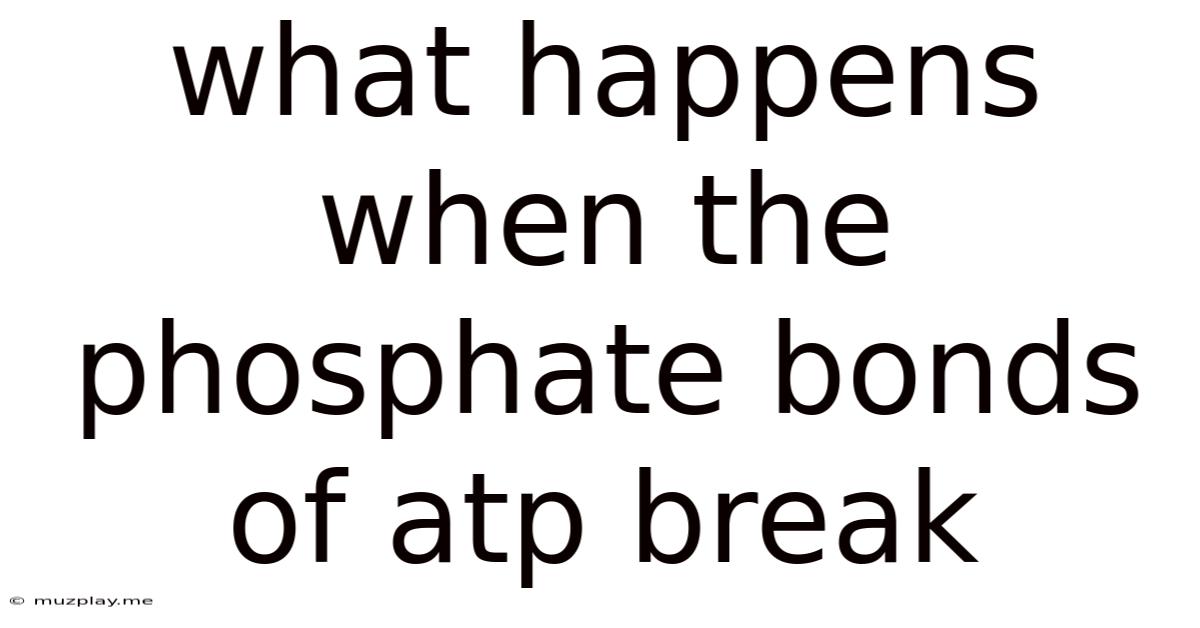
Table of Contents
What Happens When the Phosphate Bonds of ATP Break? The Energetics of Life
Adenosine triphosphate (ATP) is often called the "energy currency" of the cell. This isn't a metaphorical statement; ATP quite literally fuels the vast majority of cellular processes. Understanding what happens when the phosphate bonds of ATP break is fundamental to understanding how life itself functions. This process, hydrolysis, releases energy that drives countless reactions, from muscle contraction to protein synthesis. This article will delve deep into the intricacies of ATP hydrolysis, exploring its mechanism, the resulting energy release, and its crucial role in cellular metabolism.
The Structure of ATP: A High-Energy Molecule
Before we dissect the breaking of phosphate bonds, let's briefly examine ATP's structure. ATP is a nucleotide composed of three main components:
- Adenine: A nitrogenous base, a crucial component in DNA and RNA.
- Ribose: A five-carbon sugar molecule, providing the structural backbone.
- Three Phosphate Groups: This is where the energy magic happens. These phosphate groups are linked by high-energy phosphoanhydride bonds. It's these bonds that hold the key to ATP's energy-releasing capabilities.
The negative charges on the phosphate groups repel each other strongly. This electrostatic repulsion creates significant instability within the molecule, making the bonds relatively easy to break. This instability is the source of the high energy stored within ATP. This stored energy isn't inherently within the bonds themselves, but rather represents the potential energy released when the bonds are broken and the system moves to a lower-energy state.
ATP Hydrolysis: The Breaking of Phosphate Bonds
The process of breaking a phosphate bond in ATP is called hydrolysis. Hydrolysis involves the addition of a water molecule (H₂O) to the bond, breaking it apart. Specifically, in ATP hydrolysis, the terminal phosphate bond (the bond between the second and third phosphate groups) is typically broken. This reaction yields:
- Adenosine diphosphate (ADP): ATP minus one phosphate group.
- Inorganic phosphate (Pi): A free phosphate ion.
- Energy: This is the crucial byproduct that drives cellular processes.
The reaction can be summarized as follows:
ATP + H₂O → ADP + Pi + Energy
The energy released isn't a free-floating entity; it's harnessed to drive other reactions that require energy input. This coupling of ATP hydrolysis to energy-requiring reactions is vital for cellular function.
The Energy Released: Understanding Gibbs Free Energy
The energy released during ATP hydrolysis is often described in terms of Gibbs Free Energy (ΔG). Gibbs Free Energy represents the amount of energy available to do work at a constant temperature and pressure. For ATP hydrolysis under standard conditions (25°C, 1 atm pressure, and 1M concentration of reactants and products), the ΔG is approximately -30.5 kJ/mol. The negative sign indicates that the reaction releases energy, making it exergonic.
It's crucial to note that the actual ΔG in a living cell can vary significantly depending on the intracellular concentrations of ATP, ADP, and Pi. These concentrations are constantly regulated within a narrow range to ensure optimal energy transfer. Under cellular conditions, the ΔG is typically closer to -50 kJ/mol, reflecting the higher efficiency of energy transfer within the biological context.
Coupling ATP Hydrolysis to Cellular Processes
The energy released from ATP hydrolysis is not directly used to power cellular processes. Instead, it's coupled to other reactions, making them energetically favorable. This coupling often involves the transfer of a phosphate group from ATP to another molecule, a process known as phosphorylation. Phosphorylation can:
- Change the shape of a protein: This is critical for enzymes, altering their activity and allowing them to catalyze specific reactions. Think of it like a molecular switch being flipped.
- Increase the reactivity of a molecule: Phosphorylation can make a molecule more likely to participate in a specific reaction, lowering the activation energy required.
- Drive transport processes: The energy from ATP hydrolysis can fuel active transport systems, moving molecules against their concentration gradients across cell membranes.
Let's consider a few examples:
- Muscle Contraction: Myosin, a motor protein, utilizes ATP hydrolysis to generate the force required for muscle contraction. The phosphate group released from ATP induces a conformational change in myosin, allowing it to bind to actin and pull on it.
- Protein Synthesis: The ribosome, the protein-synthesizing machinery of the cell, requires ATP for its various functions, including the binding of tRNA molecules carrying amino acids.
- Active Transport: Sodium-potassium pumps embedded in cell membranes use ATP hydrolysis to maintain the sodium and potassium gradients crucial for nerve impulse transmission and other cellular functions.
- DNA Replication: The process of replicating DNA requires numerous enzymes and proteins, and ATP hydrolysis provides the energy necessary for these reactions.
Regeneration of ATP: A Continuous Cycle
ATP is constantly being used and regenerated within the cell. The continuous cycle of ATP hydrolysis and resynthesis is essential for maintaining the cell's energy balance. ATP regeneration predominantly occurs through:
- Cellular Respiration: This complex process in mitochondria breaks down glucose and other fuel molecules, releasing energy to synthesize ATP from ADP and Pi.
- Photosynthesis: In plants, the energy from sunlight is used to drive ATP synthesis.
The balance between ATP hydrolysis and resynthesis is tightly regulated. If the rate of ATP hydrolysis exceeds the rate of regeneration, the cell will experience an energy deficit, potentially leading to cellular dysfunction or even cell death.
ATP Hydrolysis and Disease
Dysfunction in ATP synthesis or hydrolysis is implicated in a range of diseases. Mitochondrial diseases, for example, often result from defects in the mitochondrial electron transport chain, which is crucial for ATP production. This can lead to a wide array of symptoms, depending on which tissues are most affected. Similarly, disruptions in cellular processes that rely on ATP hydrolysis can lead to various health problems.
Conclusion: The Central Role of ATP Hydrolysis in Life
The breaking of phosphate bonds in ATP is a fundamental process supporting virtually all aspects of cellular life. The energy released from ATP hydrolysis fuels countless reactions that drive cellular processes, from the simplest metabolic pathways to the most complex physiological functions. Understanding the intricate mechanisms of ATP hydrolysis is essential not only for comprehending cellular biology but also for developing therapies for diseases related to energy metabolism. The seemingly simple act of breaking a phosphate bond is, in reality, a cornerstone of life itself. Further research continues to unravel the complexities of this vital process, constantly revealing new insights into the intricate machinery of living cells. The more we learn, the more we appreciate the elegance and efficiency of this fundamental energy transaction.
Latest Posts
Latest Posts
-
Characteristics Of Ecosystems With High Biodiversity
May 12, 2025
-
Is Membrane Bound Organelles Prokaryotic Or Eukaryotic
May 12, 2025
-
Label The Structures Surrounding A Late 4 Week Old Embryo
May 12, 2025
-
Chemical Changes Involve The Breaking And Making Of Chemical Bonds
May 12, 2025
-
Solids Have Definite Shape And Volume
May 12, 2025
Related Post
Thank you for visiting our website which covers about What Happens When The Phosphate Bonds Of Atp Break . We hope the information provided has been useful to you. Feel free to contact us if you have any questions or need further assistance. See you next time and don't miss to bookmark.