What Is A Base In Biology
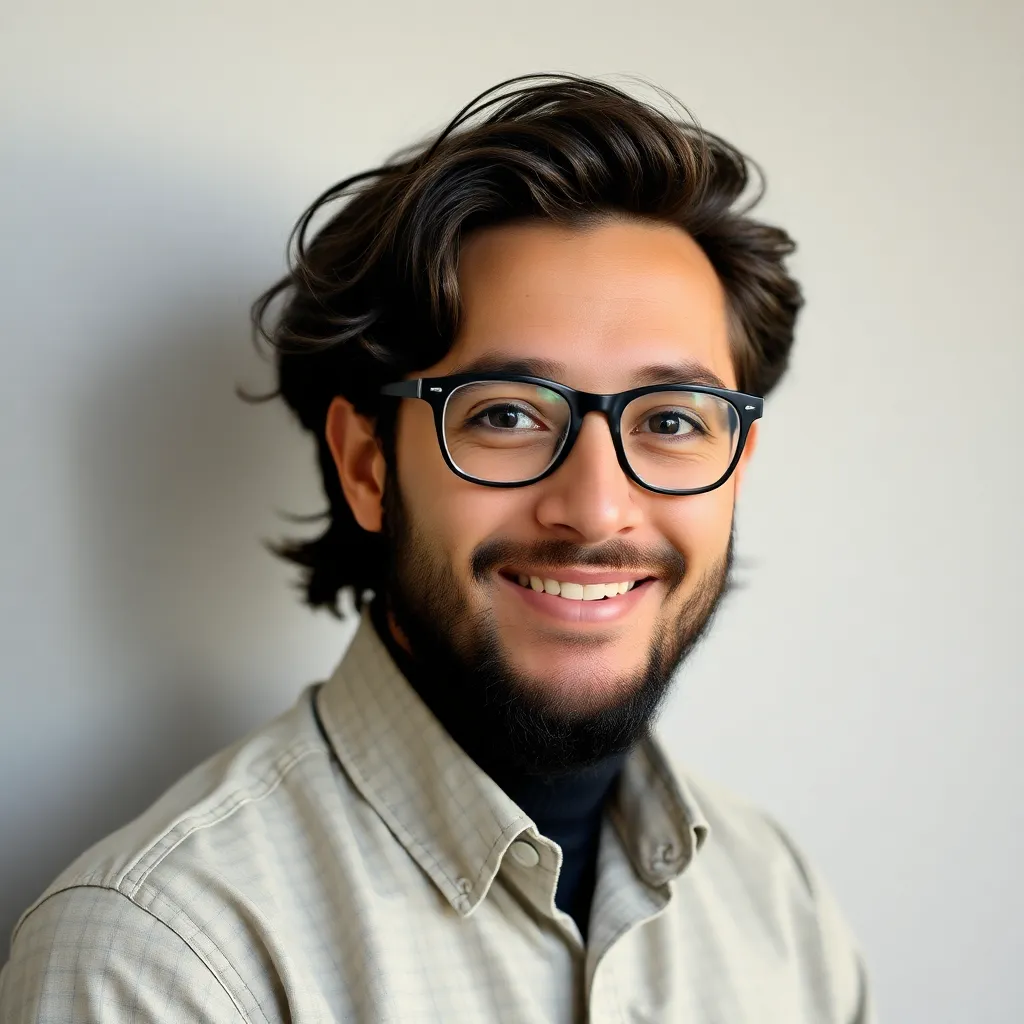
Muz Play
Mar 13, 2025 · 7 min read

Table of Contents
What is a Base in Biology? Understanding the Fundamental Building Blocks of Life
Bases, in the context of biology, are fundamental components of nucleic acids, the molecules that carry genetic information within all living organisms. Understanding what a base is and how it functions is crucial to grasping the intricacies of DNA, RNA, and the processes of heredity, gene expression, and protein synthesis. This comprehensive guide delves into the world of biological bases, exploring their structure, function, and significance in the grand scheme of life.
The Chemical Nature of Bases: Purines and Pyrimidines
Biological bases are nitrogenous compounds, meaning they contain nitrogen atoms within their ring structures. These rings are either single or double, and this structural difference defines two main categories: purines and pyrimidines.
Purines: The Double-Ringed Structures
Purines are characterized by a double-ring structure, consisting of a six-membered ring fused to a five-membered ring. In the context of nucleic acids, the two crucial purines are:
-
Adenine (A): Adenine is a crucial purine base found in both DNA and RNA. Its structure includes a six-membered ring with a nitrogen atom at positions 1 and 3, and a five-membered ring with nitrogen at position 7. It plays a vital role in energy transfer (as part of ATP), and forms base pairs with thymine (in DNA) and uracil (in RNA).
-
Guanine (G): Guanine, another purine present in both DNA and RNA, possesses a similar double-ring structure but with an additional oxygen atom at position 6. This oxygen atom contributes to its unique chemical properties. Guanine forms base pairs with cytosine in both DNA and RNA.
Pyrimidines: The Single-Ringed Structures
Pyrimidines are characterized by their single, six-membered ring structure. The key pyrimidine bases in nucleic acids are:
-
Cytosine (C): Cytosine is found in both DNA and RNA. Its single ring contains nitrogen atoms at positions 1 and 3, and an amino group at position 4. It forms a base pair with guanine.
-
Thymine (T): Thymine is a pyrimidine base found exclusively in DNA. Its structure is similar to cytosine but with an additional methyl group at position 5. It forms a base pair with adenine.
-
Uracil (U): Uracil is a pyrimidine base found exclusively in RNA. It replaces thymine in RNA and has a very similar structure, lacking the methyl group present in thymine. Uracil also pairs with adenine.
Base Pairing: The Foundation of Genetic Information
The specific arrangement of bases within DNA and RNA is fundamental to their function. Bases do not pair randomly; instead, they adhere to specific base-pairing rules, driven by hydrogen bonding between the nitrogenous bases:
-
Adenine (A) pairs with Thymine (T) in DNA (or Uracil (U) in RNA). Two hydrogen bonds form between A and T (or U).
-
Guanine (G) pairs with Cytosine (C). Three hydrogen bonds form between G and C, making this bond stronger than the A-T (or A-U) bond.
This precise base pairing is crucial for the double-helix structure of DNA, and the various secondary structures of RNA. It is also vital for the replication and transcription of genetic information. The sequence of bases along a DNA or RNA molecule encodes the genetic information, determining the amino acid sequence of proteins.
The Role of Bases in Nucleic Acid Structure and Function
The sequence of bases in DNA determines the genetic code, which is ultimately translated into proteins. Understanding this relationship is crucial to understanding the mechanics of life:
DNA: The Blueprint of Life
Deoxyribonucleic acid (DNA) utilizes the bases adenine, guanine, cytosine, and thymine to store genetic information. The double-helix structure of DNA, stabilized by base pairing and hydrophobic interactions, protects this crucial information. The sequence of bases along one strand dictates the sequence of bases on the complementary strand, facilitating accurate DNA replication and ensuring genetic fidelity.
RNA: The Messenger and Catalyst
Ribonucleic acid (RNA) uses adenine, guanine, cytosine, and uracil. RNA plays diverse roles in gene expression, including:
-
Messenger RNA (mRNA): Carries genetic information from DNA to ribosomes, where protein synthesis occurs. The sequence of mRNA bases dictates the amino acid sequence of the resulting protein.
-
Transfer RNA (tRNA): Delivers specific amino acids to the ribosome during protein synthesis. The base sequence of tRNA is crucial for recognizing the corresponding mRNA codon.
-
Ribosomal RNA (rRNA): A structural component of ribosomes, the cellular machinery responsible for protein synthesis.
Modifications of Bases: Expanding the Functional Repertoire
While the standard bases (A, G, C, T, and U) are essential, many bases undergo modifications after their incorporation into nucleic acids. These modifications can alter the structure, function, and stability of DNA and RNA molecules. Examples include:
-
Methylation: The addition of a methyl group (-CH3) to a base, commonly occurring in DNA and affecting gene expression. DNA methylation patterns can be inherited and play a critical role in epigenetic regulation.
-
Other Modifications: Various other chemical groups can be added to bases, altering their properties and affecting RNA structure and function, particularly in RNA molecules like tRNA and rRNA.
These modifications dramatically expand the functional versatility of nucleic acids, allowing for fine-tuned regulation of gene expression and a greater degree of complexity in cellular processes.
The Significance of Bases in Biological Processes
The role of bases extends far beyond the simple storage of genetic information. They are fundamental to several crucial biological processes:
DNA Replication: Faithful Copying of Genetic Information
During DNA replication, the double helix unwinds, and each strand serves as a template for the synthesis of a new complementary strand. The precise base pairing rules ensure that the newly synthesized DNA molecules are identical to the original. Errors during this process, leading to mutations in the base sequence, can have significant consequences.
Transcription: From DNA to RNA
Transcription is the process of synthesizing RNA from a DNA template. RNA polymerase, an enzyme, reads the DNA sequence and synthesizes a complementary RNA molecule. Again, precise base pairing is crucial for accurate transcription.
Translation: From RNA to Protein
Translation is the process of protein synthesis, where the genetic information encoded in mRNA is used to assemble a polypeptide chain. Transfer RNA (tRNA) molecules, carrying specific amino acids, recognize corresponding codons (three-base sequences) in the mRNA. The accuracy of this process heavily relies on the precise base pairing between mRNA codons and tRNA anticodons.
Gene Regulation: Controlling Gene Expression
The expression of genes, or the production of proteins from those genes, can be regulated at multiple levels. Modifications of bases, such as DNA methylation, play a significant role in controlling gene expression, influencing the accessibility of DNA to the transcriptional machinery.
Evolution and Adaptation: Driving the Diversity of Life
Changes in the base sequence of DNA, resulting from mutations, are the raw material for evolution. These changes can alter protein function, impacting an organism's phenotype and potentially leading to adaptations that enhance survival and reproduction. The accumulation of these changes over time drives the evolution of new species.
Base Analogues and Their Applications
Base analogues are synthetic molecules that resemble natural bases but have slightly different chemical structures. These analogues can be used in various research applications, including:
-
Studying DNA Replication and Repair: Base analogues can be incorporated into DNA during replication, providing insights into these crucial processes.
-
Developing Anticancer Drugs: Some base analogues can inhibit DNA replication in rapidly dividing cancer cells, making them useful as anticancer agents.
-
Gene Therapy: Base analogues can be used in gene editing techniques, such as CRISPR-Cas9, to precisely target and modify specific DNA sequences.
Conclusion: The Undeniable Importance of Bases in Biology
From the double helix of DNA to the complex machinery of protein synthesis, bases are the foundational components of life's information systems. Their precise pairing, structural diversity, and potential for modification contribute to the incredible complexity and adaptability of living organisms. Understanding the structure, function, and significance of bases is paramount to appreciating the intricate mechanisms of heredity, gene expression, and the very essence of life itself. Further research into base modifications and their regulatory roles will undoubtedly continue to unravel the intricate secrets encoded within these fundamental building blocks of life.
Latest Posts
Latest Posts
-
Find The Singular Values Of
May 09, 2025
-
Similarities Between The Nervous System And The Endocrine System
May 09, 2025
-
All Organic Molecules Contain The Element Carbon
May 09, 2025
-
How Doping Is Done In Semiconductor
May 09, 2025
-
Which Process Can Separate Out The Solute From The Solvent
May 09, 2025
Related Post
Thank you for visiting our website which covers about What Is A Base In Biology . We hope the information provided has been useful to you. Feel free to contact us if you have any questions or need further assistance. See you next time and don't miss to bookmark.