What Is Conduction Band And Valence Band
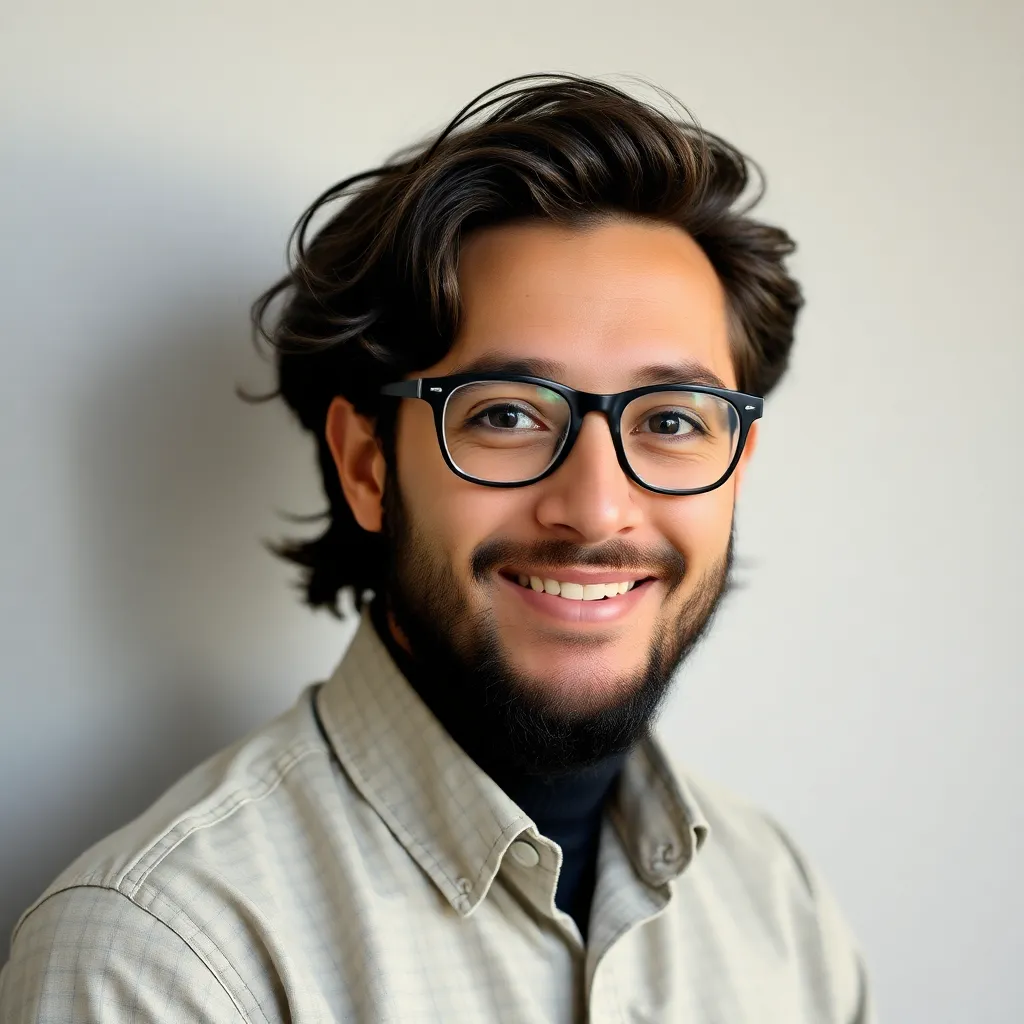
Muz Play
Apr 15, 2025 · 6 min read

Table of Contents
What is Conduction Band and Valence Band? A Deep Dive into Semiconductor Physics
Understanding the behavior of electrons within a material is crucial to comprehending its electrical properties. This understanding hinges on the concept of energy bands, specifically the conduction band and the valence band. These bands, and the energy gap between them, dictate whether a material acts as an insulator, semiconductor, or conductor. This comprehensive guide will delve into the intricacies of conduction and valence bands, exploring their significance in various applications.
Understanding Energy Bands in Solids
Before diving into the specifics of conduction and valence bands, let's establish a foundational understanding of energy bands within a solid material. Atoms possess discrete energy levels that their electrons occupy. However, when atoms come together to form a solid, these discrete energy levels broaden and overlap, forming continuous energy bands. This broadening arises from the interactions between neighboring atoms.
The Pauli Exclusion Principle dictates that each energy level can only accommodate a limited number of electrons (two electrons with opposite spins). As a result, electrons fill the available energy levels starting from the lowest energy level. At absolute zero temperature, all electrons occupy the lowest available energy states.
The energy bands are separated by forbidden energy gaps, regions where no electron states exist. The behavior of a material – whether it's a conductor, insulator, or semiconductor – is directly influenced by the arrangement and occupancy of these energy bands.
The Valence Band: A Sea of Bound Electrons
The valence band is the highest energy band that is completely filled with electrons at absolute zero temperature. The electrons in the valence band are tightly bound to their respective atoms and are responsible for the material's chemical bonding. These electrons do not contribute to the material's electrical conductivity at low temperatures because they lack sufficient energy to move freely throughout the material.
Understanding Valence Band Properties:
- Electron Occupancy: Completely filled at absolute zero.
- Electron Mobility: Low mobility due to strong atomic bonds.
- Contribution to Conductivity: Minimal contribution to conductivity at low temperatures.
- Band Structure Variation: The shape and width of the valence band vary depending on the material's crystal structure and atomic composition. For example, silicon and germanium, common semiconductors, have slightly different valence band structures.
The Conduction Band: The Highway for Electron Movement
The conduction band is the energy band above the valence band. Crucially, at absolute zero temperature, it is typically empty or nearly empty. Electrons in the conduction band are loosely bound to the atoms and possess sufficient energy to move freely throughout the material's crystal lattice. This free movement is what enables electrical conduction.
Understanding Conduction Band Properties:
- Electron Occupancy: Typically empty or partially filled at absolute zero.
- Electron Mobility: High mobility due to weak atomic bonds.
- Contribution to Conductivity: Directly responsible for electrical conductivity.
- Band Structure Variation: Similar to the valence band, the conduction band's structure varies between materials. The shape and energy level play a role in determining the material’s electrical properties.
The Energy Band Gap: The Deciding Factor
The crucial difference between conductors, insulators, and semiconductors lies in the energy band gap (Eg) – the energy difference between the top of the valence band and the bottom of the conduction band. This band gap determines the ease with which electrons can transition from the valence band to the conduction band.
Conductors: No Gap, Abundant Conductivity
In conductors, the valence band and conduction band overlap, or there is a very small band gap. This means that electrons can easily transition to the conduction band, even at absolute zero temperature. This results in a high concentration of charge carriers and excellent electrical conductivity. Examples include metals like copper and silver.
Insulators: Large Gap, Minimal Conductivity
Insulators possess a large energy band gap (typically greater than 3 eV). This significant energy barrier prevents electrons from easily transitioning from the valence band to the conduction band at room temperature. Therefore, insulators exhibit very low electrical conductivity. Examples include diamond and glass.
Semiconductors: Bridging the Gap
Semiconductors have a moderate energy band gap (typically between 0.1 eV and 3 eV). At absolute zero, they behave like insulators. However, at higher temperatures or with the addition of impurities (doping), some electrons can gain enough energy to jump from the valence band to the conduction band. This allows for controlled electrical conductivity, making semiconductors essential in electronic devices. Silicon and germanium are classic examples.
Doping: Modifying Semiconductor Properties
The electrical properties of semiconductors can be significantly altered through a process called doping. Doping involves introducing impurity atoms with a different number of valence electrons than the host semiconductor atoms. This creates either an excess of electrons (n-type doping) or an absence of electrons (holes) in the valence band (p-type doping).
N-Type Doping: Increasing Electrons
N-type doping involves introducing impurity atoms with more valence electrons than the host semiconductor atoms (e.g., phosphorus in silicon). These extra electrons occupy the conduction band, significantly increasing the conductivity. The impurity atoms are known as donor atoms because they donate electrons to the conduction band.
P-Type Doping: Increasing Holes
P-type doping involves introducing impurity atoms with fewer valence electrons than the host semiconductor atoms (e.g., boron in silicon). This creates "holes" – vacancies in the valence band – which behave like positively charged carriers. These holes can move through the crystal lattice, contributing to conductivity. The impurity atoms are known as acceptor atoms because they accept electrons from the valence band, creating holes.
Applications of Conduction and Valence Bands
The concepts of conduction and valence bands are fundamental to understanding a vast array of electronic and optoelectronic devices. Their properties are exploited in numerous technologies:
- Transistors: The foundation of modern electronics, transistors rely on the controlled flow of electrons between n-type and p-type semiconductor regions.
- Integrated Circuits (ICs): Millions of transistors are integrated onto a single chip to create complex electronic circuits, enabling the functionality of computers, smartphones, and other digital devices.
- Diodes: Diodes allow current to flow in one direction only, based on the p-n junction formed by combining p-type and n-type semiconductors.
- Solar Cells: Solar cells utilize the photovoltaic effect, where photons excite electrons from the valence band to the conduction band, generating an electrical current.
- Light Emitting Diodes (LEDs): LEDs emit light when electrons in the conduction band recombine with holes in the valence band, releasing energy as photons.
- Lasers: Similar to LEDs, lasers rely on stimulated emission of light when electrons recombine in the conduction and valence band, producing coherent light.
Advanced Concepts and Further Exploration
This article provides a foundational understanding of conduction and valence bands. Further exploration could delve into:
- Effective mass: The concept of effective mass considers how electrons behave differently in a crystal lattice compared to free electrons.
- Density of states: The number of available energy states within a given energy range.
- Fermi level: The highest occupied energy level at absolute zero temperature.
- Band diagrams: Visual representations of the energy band structure of materials.
- Carrier mobility and lifetime: Key factors influencing semiconductor performance.
Conclusion: The Heart of Semiconductor Physics
The conduction and valence bands are fundamental concepts in semiconductor physics and materials science. Understanding their properties, the energy band gap, and the impact of doping is crucial for designing and developing advanced electronic and optoelectronic devices. This deep dive highlights the critical role these bands play in shaping the technological landscape around us. Further exploration into the advanced concepts mentioned above will provide a more comprehensive understanding of the behavior of electrons in solids and the exciting possibilities for future technological advancements.
Latest Posts
Latest Posts
-
The Chemical Reaction That Creates Polymers Is Called
May 09, 2025
-
The Variety Of Biotic And Abiotic Factors In An Ecosystem
May 09, 2025
-
How To Write A Polynomial Function From A Graph
May 09, 2025
-
How Many Phosphates Would Adp Have Attached To It
May 09, 2025
-
Which Of The Following Series Is Absolutely Convergent
May 09, 2025
Related Post
Thank you for visiting our website which covers about What Is Conduction Band And Valence Band . We hope the information provided has been useful to you. Feel free to contact us if you have any questions or need further assistance. See you next time and don't miss to bookmark.