What Is Required For Glucose Catabolism To Proceed Beyond Glycolysis
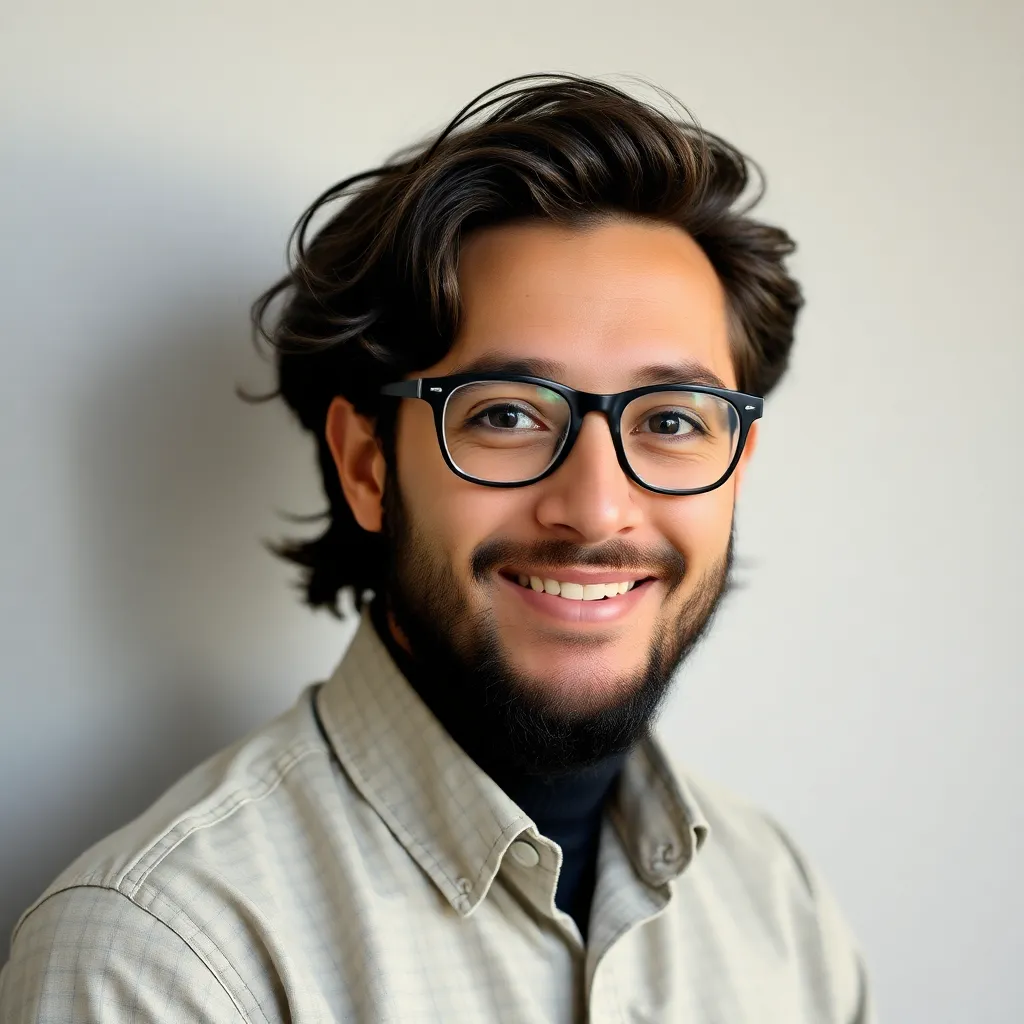
Muz Play
May 10, 2025 · 7 min read
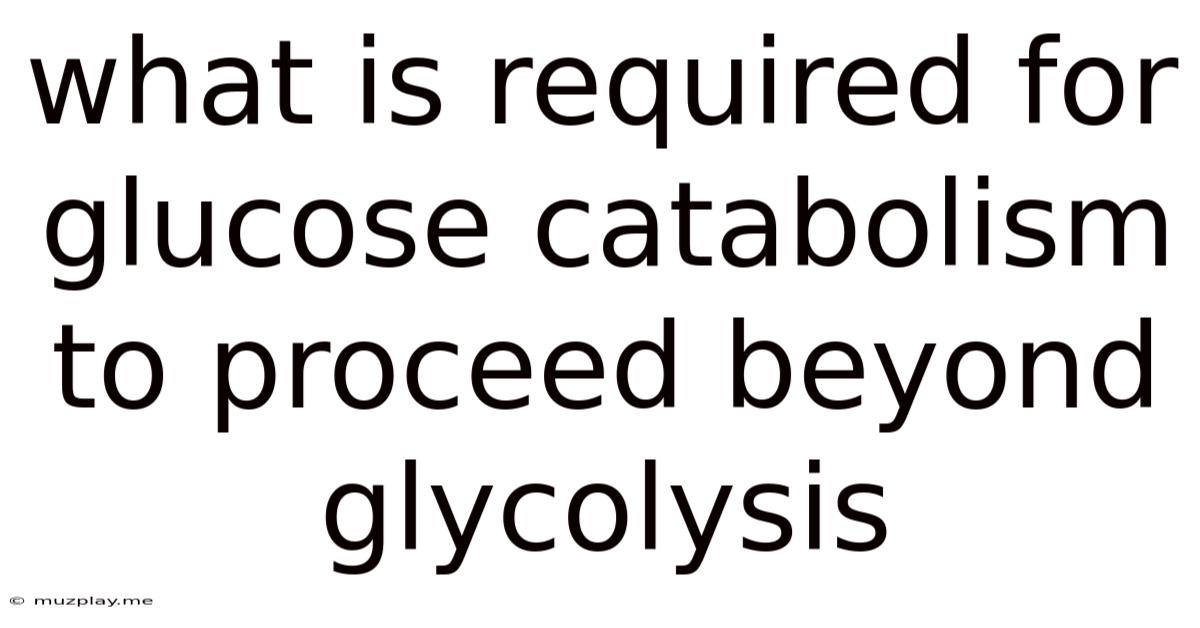
Table of Contents
What is Required for Glucose Catabolism to Proceed Beyond Glycolysis?
Glucose catabolism, the process of breaking down glucose to generate energy, is a vital metabolic pathway for all living organisms. Glycolysis, the first stage of this process, occurs in the cytoplasm and yields a small amount of ATP (adenosine triphosphate), the cell's energy currency. However, to fully harness the energy stored within glucose, catabolism must proceed beyond glycolysis. This requires a series of intricate steps, involving specific enzymes, coenzymes, and cellular compartments, all finely tuned to maximize energy production. This article will delve into the requirements for glucose catabolism to proceed beyond glycolysis, examining the pivotal roles of pyruvate oxidation, the citric acid cycle (Krebs cycle), and oxidative phosphorylation.
The Transition: Pyruvate Oxidation – Bridging Glycolysis and the Citric Acid Cycle
Glycolysis's end product, pyruvate, is a three-carbon molecule that isn't directly compatible with the next stage of glucose catabolism. Before entering the citric acid cycle, pyruvate must undergo pyruvate oxidation, a crucial transition step occurring within the mitochondrial matrix. This process requires several key components:
1. Pyruvate Dehydrogenase Complex (PDC): The Orchestrator
The central player in pyruvate oxidation is the pyruvate dehydrogenase complex (PDC), a multi-enzyme complex that catalyzes the irreversible conversion of pyruvate to acetyl-CoA. This complex requires five coenzymes for its activity:
- Thiamine pyrophosphate (TPP): A derivative of vitamin B1, TPP plays a crucial role in decarboxylation, removing a carbon dioxide molecule from pyruvate.
- Lipoic acid: A cofactor that acts as an acyl group carrier, transferring the acetyl group from pyruvate to Coenzyme A.
- Coenzyme A (CoA): A crucial coenzyme that carries the acetyl group to the citric acid cycle.
- Flavin adenine dinucleotide (FAD): An oxidized form of a riboflavin-derived coenzyme, accepting electrons during the oxidation process.
- Nicotinamide adenine dinucleotide (NAD+): Another crucial coenzyme that accepts electrons, becoming reduced to NADH.
The availability of these coenzymes is essential for PDC function. Deficiencies in any of the B vitamins required for their synthesis can significantly impair pyruvate oxidation, leading to metabolic disorders.
2. Mitochondrial Transport: Getting Pyruvate into the Matrix
Pyruvate, produced in the cytoplasm during glycolysis, must be transported into the mitochondrial matrix to undergo oxidation. This transport is facilitated by specific mitochondrial pyruvate carriers, which actively transport pyruvate across the inner mitochondrial membrane. The efficiency of this transport is crucial, as it dictates the rate at which pyruvate can be processed.
3. Oxygen: The Ultimate Electron Acceptor (Indirectly)
While not directly involved in pyruvate oxidation itself, the presence of oxygen is indirectly crucial. The NADH produced during pyruvate oxidation will ultimately donate its electrons to the electron transport chain (ETC) in oxidative phosphorylation, a process that requires oxygen as the final electron acceptor. Without oxygen, the ETC would become stalled, and NADH regeneration would cease, halting pyruvate oxidation.
The Citric Acid Cycle (Krebs Cycle): Central Hub of Metabolism
The acetyl-CoA produced from pyruvate oxidation enters the citric acid cycle (CAC), also known as the Krebs cycle or tricarboxylic acid (TCA) cycle. This cycle is a central metabolic hub, not only involved in glucose catabolism but also in the oxidation of fatty acids and amino acids. Several essential components are required for the efficient functioning of the CAC:
1. Oxaloacetate: The Cycle's Starter Molecule
The citric acid cycle begins with the condensation of acetyl-CoA with oxaloacetate, a four-carbon molecule, to form citrate (citric acid), a six-carbon molecule. Oxaloacetate is regenerated at the end of the cycle, ensuring its continuous operation. The availability of oxaloacetate is therefore crucial for the smooth functioning of the CAC. Several metabolic pathways contribute to oxaloacetate production, highlighting the interconnectedness of metabolic processes.
2. Enzymes: Catalyzing Each Step
The citric acid cycle comprises a series of eight enzyme-catalyzed reactions. Each step requires specific enzymes, including citrate synthase, aconitase, isocitrate dehydrogenase, α-ketoglutarate dehydrogenase, succinyl-CoA synthetase, succinate dehydrogenase, fumarase, and malate dehydrogenase. The proper functioning of these enzymes is paramount for the cycle’s efficient operation. Genetic defects or deficiencies in these enzymes can lead to significant metabolic disturbances.
3. Coenzymes: Electron Carriers and Energy Transfer
The CAC generates several high-energy electron carriers:
- NADH: Produced in three steps of the cycle, NADH carries electrons to the electron transport chain.
- FADH2: Produced in one step of the cycle, FADH2 also carries electrons to the electron transport chain, although it enters at a different point than NADH.
- GTP (Guanosine triphosphate): A high-energy molecule similar to ATP, produced directly in one step of the cycle through substrate-level phosphorylation. GTP can readily be converted to ATP.
The availability of NAD+ and FAD is crucial for the continued function of the CAC. These coenzymes are regenerated during oxidative phosphorylation, linking the CAC to the final stage of glucose catabolism.
4. Mitochondrial Matrix: The Location of the Cycle
The citric acid cycle takes place exclusively within the mitochondrial matrix. This compartmentalization allows for efficient coupling between the CAC and oxidative phosphorylation, ensuring maximal energy yield.
Oxidative Phosphorylation: Harvesting the Energy
The final stage of glucose catabolism is oxidative phosphorylation, which takes place across the inner mitochondrial membrane. This process harnesses the energy stored in the electron carriers (NADH and FADH2) produced during glycolysis, pyruvate oxidation, and the citric acid cycle to generate large amounts of ATP.
1. Electron Transport Chain (ETC): A Cascade of Electron Transfers
The ETC comprises a series of protein complexes embedded in the inner mitochondrial membrane. Electrons from NADH and FADH2 are sequentially transferred through these complexes, releasing energy along the way. This energy is used to pump protons (H+) from the mitochondrial matrix to the intermembrane space, creating a proton gradient.
2. Chemiosmosis: The Proton Motive Force
The proton gradient generated by the ETC creates a proton motive force (PMF), a form of potential energy. This PMF drives the synthesis of ATP through a process called chemiosmosis. Protons flow back into the matrix through a protein complex called ATP synthase, which uses this energy to phosphorylate ADP to ATP.
3. Oxygen: The Final Electron Acceptor
Oxygen plays a crucial role in oxidative phosphorylation as the final electron acceptor in the ETC. Electrons from NADH and FADH2 are ultimately transferred to oxygen, forming water. Without oxygen, the ETC would become stalled, and ATP synthesis would cease. This explains why aerobic respiration generates significantly more ATP than anaerobic processes.
4. Mitochondrial Structure and Function: A Specialized Organelle
The mitochondrion itself is essential for oxidative phosphorylation. Its highly folded inner membrane, known as cristae, dramatically increases the surface area available for the ETC and ATP synthase, maximizing ATP production. The compartmentalization of the ETC and ATP synthase within the inner mitochondrial membrane is critical for the efficient generation of the proton gradient.
Regulation and Integration: A Highly Coordinated Process
Glucose catabolism is not simply a linear pathway; it's a highly regulated and integrated process. Several mechanisms ensure its efficient operation and coordination with other metabolic pathways:
- Feedback Inhibition: The activity of key enzymes in glycolysis, pyruvate oxidation, and the citric acid cycle is regulated by feedback inhibition, where the accumulation of end products inhibits the activity of earlier enzymes in the pathway. This prevents the overproduction of ATP and other metabolic intermediates.
- Hormonal Regulation: Hormones such as insulin and glucagon play critical roles in regulating glucose metabolism, influencing the rate of glycolysis, pyruvate oxidation, and the citric acid cycle.
- Allosteric Regulation: Many enzymes involved in glucose catabolism are subject to allosteric regulation, where the binding of small molecules (allosteric effectors) affects their activity. This allows for fine-tuning of metabolic fluxes based on cellular energy demands.
- Metabolic Interconnections: Glucose catabolism is intimately linked with other metabolic pathways, such as fatty acid oxidation and amino acid metabolism. These pathways share intermediates and regulatory mechanisms, ensuring efficient allocation of metabolic resources.
Conclusion: A Symphony of Metabolic Processes
The progression of glucose catabolism beyond glycolysis is a complex and highly regulated process requiring the coordinated action of numerous enzymes, coenzymes, and cellular compartments. Pyruvate oxidation, the citric acid cycle, and oxidative phosphorylation are essential steps that yield a significant amount of ATP from each glucose molecule. The availability of oxygen and the proper functioning of mitochondrial structures and enzymes are crucial for the efficient production of energy. Understanding these requirements provides valuable insight into the intricate workings of cellular metabolism and the importance of maintaining metabolic homeostasis. Dysfunction at any stage can lead to severe metabolic disorders, highlighting the critical importance of this pathway for life.
Latest Posts
Latest Posts
-
Do Double Bonds Increase Melting Point
May 10, 2025
-
When Is It Acceptable To Use A Personal Platform
May 10, 2025
-
Which Image Represents Cytokinesis In A Plant Cell
May 10, 2025
-
How Is Thermal Energy And Temperature Related
May 10, 2025
-
Why Can A Gas Be Compressed
May 10, 2025
Related Post
Thank you for visiting our website which covers about What Is Required For Glucose Catabolism To Proceed Beyond Glycolysis . We hope the information provided has been useful to you. Feel free to contact us if you have any questions or need further assistance. See you next time and don't miss to bookmark.