What Is The Building Block Monomer For A Nucleic Acids
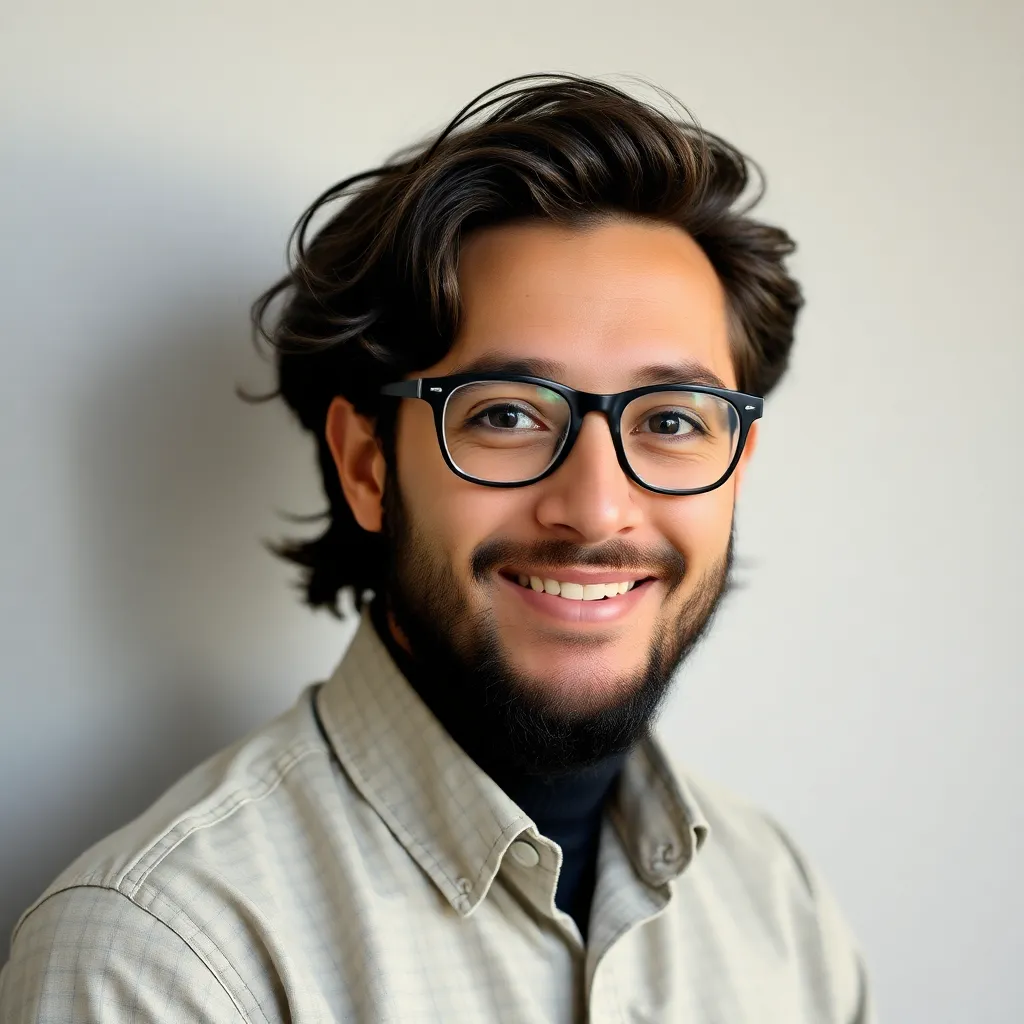
Muz Play
Apr 07, 2025 · 7 min read
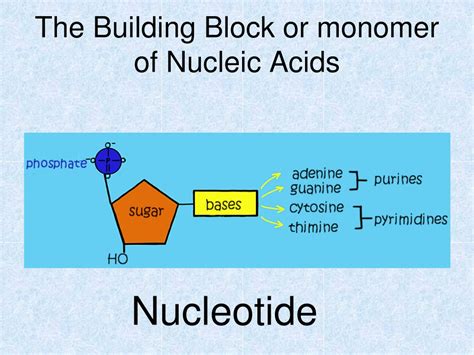
Table of Contents
What is the Building Block Monomer for Nucleic Acids?
Nucleic acids, the fundamental molecules of life, are responsible for storing and transmitting genetic information. Understanding their structure is crucial to understanding how life works. This article delves deep into the building blocks of nucleic acids, exploring their composition, function, and the intricate ways they assemble to form the magnificent macromolecules that govern heredity.
The Monomer: Nucleotides – The Essential Building Blocks
The basic building block, or monomer, of nucleic acids is the nucleotide. Think of nucleotides as the individual letters in the vast alphabet of life. These molecules are not just simple units; they are complex structures with three key components:
1. A Pentose Sugar: The Structural Backbone
The pentose sugar forms the structural backbone of the nucleotide. There are two types of pentose sugars found in nucleic acids:
- Ribose: Found in ribonucleic acid (RNA). Ribose is a five-carbon sugar with a hydroxyl (-OH) group attached to the 2' carbon atom.
- Deoxyribose: Found in deoxyribonucleic acid (DNA). Deoxyribose is also a five-carbon sugar, but it lacks the hydroxyl group on the 2' carbon atom. This seemingly small difference has profound implications for the structure and stability of DNA and RNA. The absence of the 2'-OH group in deoxyribose makes DNA more resistant to hydrolysis (breakdown by water) than RNA, which is crucial for the long-term storage of genetic information.
The difference between ribose and deoxyribose is a key factor distinguishing DNA and RNA. This seemingly small chemical variation has significant consequences for the structure, stability, and function of these two critical biomolecules. Understanding this difference is fundamental to grasping the unique roles of DNA and RNA in cellular processes.
2. A Nitrogenous Base: The Information Carrier
The nitrogenous base is the information-carrying part of the nucleotide. It is attached to the 1' carbon atom of the pentose sugar. There are five main types of nitrogenous bases:
- Adenine (A): A purine base, characterized by a double-ring structure.
- Guanine (G): Another purine base, also with a double-ring structure.
- Cytosine (C): A pyrimidine base, with a single-ring structure.
- Thymine (T): A pyrimidine base, found only in DNA.
- Uracil (U): A pyrimidine base, found only in RNA, replacing thymine.
The specific sequence of these nitrogenous bases along the nucleic acid strand determines the genetic code. The pairing of these bases (A with T or U, and G with C) through hydrogen bonds is crucial for the double helix structure of DNA and the various secondary structures of RNA. The precise arrangement of these bases dictates the genetic instructions for building and maintaining an organism. This sequence is the essence of the genetic code.
3. Phosphate Group: Linking the Nucleotides
The phosphate group is a crucial component, connecting nucleotides together to form the polynucleotide chain. It's attached to the 5' carbon atom of the pentose sugar. This phosphate group forms a phosphodiester bond with the 3' carbon atom of the adjacent nucleotide, creating the sugar-phosphate backbone of the nucleic acid molecule.
The phosphate group plays a significant role in the overall structure and properties of nucleic acids. Its negative charge contributes to the overall negative charge of DNA and RNA, influencing their interactions with proteins and other cellular components. The phosphodiester bond itself is a relatively strong covalent bond, crucial for maintaining the integrity of the nucleic acid chain. The phosphate group acts as the glue holding the nucleotide monomers together, creating the linear polymer.
From Nucleotides to Nucleic Acids: Polymerization
The process of assembling nucleotides into nucleic acids is called polymerization. It's a complex process involving enzymes and requires energy input. During polymerization:
-
Activation: Nucleotides are first activated by attaching them to a high-energy molecule, typically triphosphate nucleotides (like ATP, GTP, CTP, and UTP). This activation provides the energy needed for the formation of the phosphodiester bond.
-
Bond Formation: The activated nucleotide is then added to the growing polynucleotide chain. The 3' hydroxyl group of the last nucleotide in the chain reacts with the 5' phosphate group of the incoming nucleotide, forming a phosphodiester bond and releasing a pyrophosphate molecule. This reaction is catalyzed by enzymes called DNA polymerases (for DNA) and RNA polymerases (for RNA).
-
Chain Elongation: This process is repeated, adding nucleotides one by one to the 3' end of the growing chain, resulting in the formation of a long polynucleotide chain. The directionality of this process, from 5' to 3', is vital for understanding DNA replication and transcription.
The precise sequence of nucleotides during polymerization is dictated by several factors, including the template strand (in DNA replication and transcription), the enzyme's specificity, and the availability of the appropriate activated nucleotides. The fidelity of this process is paramount, as errors can lead to mutations with potentially devastating consequences.
The Distinctive Roles of DNA and RNA: A Tale of Two Nucleic Acids
While both DNA and RNA are built from nucleotides, their structures and functions differ significantly. These differences reflect their distinct roles in cellular processes:
DNA: The Blueprint of Life
DNA, or deoxyribonucleic acid, is primarily responsible for storing genetic information. Its double-stranded helix structure, stabilized by hydrogen bonds between complementary base pairs (A-T and G-C), provides a robust and stable repository for the vast amount of genetic data required to build and maintain an organism. The double helix also facilitates accurate replication, ensuring faithful transmission of genetic information from one generation to the next. DNA's stability is crucial for the long-term preservation of the genetic code.
RNA: The Versatile Messenger and Workhorse
RNA, or ribonucleic acid, is more versatile and functionally diverse than DNA. Several types of RNA exist, each with a distinct role:
- Messenger RNA (mRNA): Carries genetic information from DNA to ribosomes, where it serves as a template for protein synthesis.
- Transfer RNA (tRNA): Carries amino acids to the ribosome, matching them to the codons on mRNA during translation.
- Ribosomal RNA (rRNA): A structural component of ribosomes, essential for protein synthesis.
- Small nuclear RNA (snRNA): Involved in splicing pre-mRNA.
- MicroRNA (miRNA): Regulates gene expression by binding to mRNA and inhibiting translation.
RNA's single-stranded nature allows it to adopt various secondary and tertiary structures, facilitating its diverse functions. Its susceptibility to hydrolysis makes it less stable than DNA, but this also contributes to its dynamic role in cellular processes. RNA's versatility allows it to participate in a wide range of cellular functions.
The Importance of Nucleotide Structure and Function
The precise structure of nucleotides, with their specific pentose sugars, nitrogenous bases, and phosphate groups, is critical to their function. Even small variations, like the presence or absence of a hydroxyl group on the 2' carbon of the pentose sugar, have profound implications for the stability and function of the resulting nucleic acid.
The hydrogen bonding between complementary base pairs is the foundation for the double helix structure of DNA and the diverse secondary structures of RNA. This precise pairing is essential for accurate DNA replication and transcription, ensuring the faithful transmission and expression of genetic information. The interaction between bases and the specificity of their pairing are central to genetic information processing.
The phosphodiester backbone provides the structural framework for the nucleic acid polymer, defining its directionality and influencing its interactions with other molecules. The negative charge of the phosphate groups is vital for the interactions between nucleic acids and proteins, influencing processes like DNA packaging and gene regulation. The structural integrity provided by the backbone is fundamental to the overall function.
Conclusion: The Building Blocks of Life
In conclusion, nucleotides are the fundamental building blocks of nucleic acids – DNA and RNA. Their composition – a pentose sugar, a nitrogenous base, and a phosphate group – dictates their properties and functions. The precise sequence of nucleotides encodes genetic information, while their structural characteristics determine the stability and functional versatility of DNA and RNA. Understanding the structure and function of nucleotides is crucial to comprehending the intricate mechanisms of heredity, gene expression, and the very essence of life itself. The seemingly simple monomer, the nucleotide, underpins the complexity and beauty of life's genetic blueprint. Further research into the nuanced aspects of nucleotide structure and interactions continues to reveal deeper insights into the molecular mechanisms governing life processes.
Latest Posts
Latest Posts
-
Is Changing Color A Chemical Change
Apr 09, 2025
-
What Is The Sugar That Helps Make Up Atp
Apr 09, 2025
-
Which Of The Following Indicate Weakness In Phylogenetic Tree
Apr 09, 2025
-
Draw The Meso Form Of This Compound
Apr 09, 2025
-
Increases Surface Area Of Plasma Membrane For Absorption
Apr 09, 2025
Related Post
Thank you for visiting our website which covers about What Is The Building Block Monomer For A Nucleic Acids . We hope the information provided has been useful to you. Feel free to contact us if you have any questions or need further assistance. See you next time and don't miss to bookmark.