What Is The Correct Order Of Events For Cellular Respiration
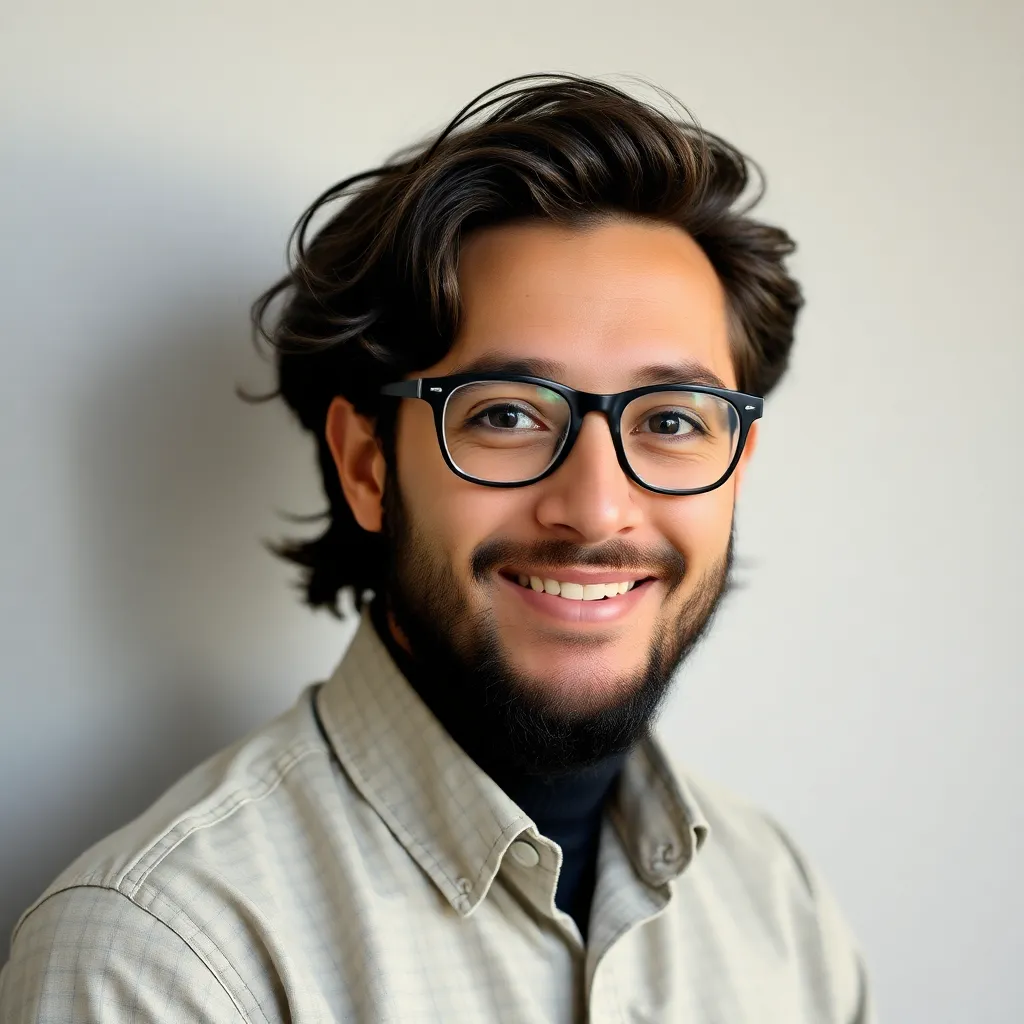
Muz Play
Apr 06, 2025 · 6 min read
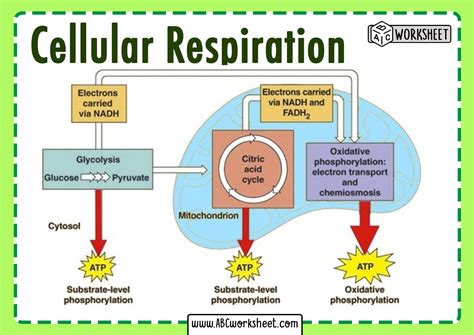
Table of Contents
What is the Correct Order of Events for Cellular Respiration?
Cellular respiration is a fundamental process in all living organisms, a complex series of metabolic reactions that convert the chemical energy stored in nutrient molecules into adenosine triphosphate (ATP), the cell's primary energy currency. Understanding the precise order of events in cellular respiration is crucial for grasping its efficiency and the intricate control mechanisms that govern it. This detailed guide will dissect each stage, clarifying the sequence and highlighting the key players involved.
The Four Stages of Cellular Respiration: A Sequential Breakdown
Cellular respiration can be broadly divided into four main stages:
- Glycolysis: The initial breakdown of glucose.
- Pyruvate Oxidation: Preparing pyruvate for the citric acid cycle.
- Citric Acid Cycle (Krebs Cycle): A central metabolic pathway generating high-energy electron carriers.
- Oxidative Phosphorylation (Electron Transport Chain & Chemiosmosis): The final stage, producing the bulk of ATP.
Let's delve into each stage sequentially, exploring the precise order of events within each step.
1. Glycolysis: The First Step in Energy Extraction
Glycolysis, meaning "sugar splitting," occurs in the cytoplasm and doesn't require oxygen. It's a ten-step process that converts one molecule of glucose (a six-carbon sugar) into two molecules of pyruvate (a three-carbon compound).
Key Events in Glycolysis:
- Phosphorylation: Glucose is phosphorylated twice, consuming two ATP molecules. This creates a highly reactive molecule that is trapped within the cell.
- Cleavage: The six-carbon sugar is split into two three-carbon molecules, glyceraldehyde-3-phosphate (G3P).
- Oxidation and Phosphorylation: Each G3P molecule is oxidized, releasing electrons that are picked up by NAD+ to form NADH. Inorganic phosphate is also added, producing ATP via substrate-level phosphorylation.
- Pyruvate Formation: The resulting molecules are converted into pyruvate.
Net yield of glycolysis: 2 ATP (net gain, after the initial investment of 2 ATP), 2 NADH, and 2 pyruvate molecules. While seemingly a small ATP yield, glycolysis is vital because it provides the starting material for subsequent stages and can function under anaerobic conditions.
2. Pyruvate Oxidation: Preparing for the Citric Acid Cycle
Before pyruvate can enter the citric acid cycle, it must undergo oxidative decarboxylation in the mitochondrial matrix. This transition stage links glycolysis to the subsequent oxidative processes.
Key Events in Pyruvate Oxidation:
- Transport: Pyruvate is transported from the cytoplasm into the mitochondrial matrix.
- Decarboxylation: A carbon atom is removed from pyruvate as carbon dioxide (CO2).
- Oxidation: The remaining two-carbon fragment is oxidized, and the electrons are transferred to NAD+, forming NADH.
- Acetyl-CoA Formation: The two-carbon fragment is attached to coenzyme A (CoA), forming acetyl-CoA. Acetyl-CoA is the molecule that enters the citric acid cycle.
Yield per pyruvate molecule: 1 NADH, 1 CO2, and 1 acetyl-CoA. Since two pyruvate molecules are produced per glucose molecule, the total yield from this stage is double these values.
3. The Citric Acid Cycle (Krebs Cycle): The Central Metabolic Hub
The citric acid cycle, a cyclic pathway occurring in the mitochondrial matrix, is a central metabolic hub that plays a crucial role in energy production and various metabolic pathways. Acetyl-CoA from pyruvate oxidation enters the cycle, undergoing a series of reactions that generate high-energy electron carriers and release CO2.
Key Events in the Citric Acid Cycle:
- Citrate Synthesis: Acetyl-CoA combines with oxaloacetate to form citrate (a six-carbon molecule).
- Isomerization and Oxidations: Citrate undergoes a series of isomerizations and oxidations, releasing CO2 and generating NADH and FADH2 (another electron carrier).
- Substrate-Level Phosphorylation: One ATP molecule is generated through substrate-level phosphorylation.
- Regeneration of Oxaloacetate: The cycle concludes with the regeneration of oxaloacetate, preparing for another round.
Yield per acetyl-CoA molecule: 3 NADH, 1 FADH2, 1 ATP, and 2 CO2. Since two acetyl-CoA molecules are produced per glucose molecule, the total yield from the citric acid cycle is double these values.
4. Oxidative Phosphorylation: ATP Synthesis through Electron Transport and Chemiosmosis
Oxidative phosphorylation, the final stage, occurs in the inner mitochondrial membrane and is responsible for the majority of ATP production during cellular respiration. It involves two closely coupled processes: the electron transport chain and chemiosmosis.
The Electron Transport Chain (ETC):
The ETC consists of a series of protein complexes embedded in the inner mitochondrial membrane. Electrons from NADH and FADH2, generated during previous stages, are passed down the chain, releasing energy. This energy is used to pump protons (H+) from the mitochondrial matrix across the inner membrane into the intermembrane space, establishing a proton gradient.
Chemiosmosis: ATP Synthase and the Proton Motive Force
The proton gradient created by the ETC represents a form of stored energy, referred to as the proton motive force. Protons flow back down their concentration gradient, from the intermembrane space to the matrix, through a protein complex called ATP synthase. This flow drives the synthesis of ATP from ADP and inorganic phosphate (Pi), a process called chemiosmosis. This is oxidative phosphorylation because the energy for ATP synthesis comes from the oxidation of electron carriers.
Calculating ATP Yield from Oxidative Phosphorylation:
The exact ATP yield from oxidative phosphorylation varies slightly depending on the shuttle system used to transport electrons from NADH in the cytoplasm to the mitochondria. However, a common estimate is approximately:
- Each NADH generates approximately 2.5 ATP molecules.
- Each FADH2 generates approximately 1.5 ATP molecules.
Considering the total NADH and FADH2 produced during glycolysis, pyruvate oxidation, and the citric acid cycle, the theoretical maximum ATP yield from oxidative phosphorylation is substantial.
Total ATP Yield: A Summary of Cellular Respiration's Energy Harvest
The total ATP yield from cellular respiration varies slightly depending on the shuttle system used and the efficiency of the process. However, a commonly cited estimate is around 30-32 ATP molecules per glucose molecule. This includes the net ATP gain from glycolysis (2 ATP), the ATP from substrate-level phosphorylation in the citric acid cycle (2 ATP), and the ATP generated through oxidative phosphorylation.
Regulation of Cellular Respiration: Maintaining Metabolic Balance
Cellular respiration is tightly regulated to meet the energy demands of the cell while avoiding wasteful energy expenditure. Several factors influence the rate of respiration, including:
- ATP levels: High ATP levels inhibit key enzymes in glycolysis and the citric acid cycle.
- NADH and FADH2 levels: High levels of these electron carriers also inhibit certain enzymes.
- Oxygen availability: Oxygen is the final electron acceptor in the electron transport chain. Its absence drastically reduces ATP production.
- Substrate availability: The availability of glucose and other fuel molecules affects the rate of respiration.
Conclusion: The Precise Orchestration of Cellular Respiration
The correct order of events in cellular respiration is critical for efficient energy production. The four stages—glycolysis, pyruvate oxidation, the citric acid cycle, and oxidative phosphorylation—work in a precisely orchestrated sequence, with each stage providing essential intermediates and electron carriers for the next. Understanding this intricate process is crucial for comprehending cellular metabolism, energy balance, and the fundamental processes that sustain life. The high ATP yield from cellular respiration, coupled with its tight regulation, ensures that cells have the energy they need to perform their diverse functions.
Latest Posts
Latest Posts
-
Test Statistic For Hypothesis Test Calculator
Apr 06, 2025
-
What Is The Driving Force For An Acid Base Neutralization Reaction
Apr 06, 2025
-
How To Find Basis Of A Subspace
Apr 06, 2025
-
How To Tell If A Transformation Is Linear
Apr 06, 2025
-
How To Find The Kernel Of A Linear Transformation
Apr 06, 2025
Related Post
Thank you for visiting our website which covers about What Is The Correct Order Of Events For Cellular Respiration . We hope the information provided has been useful to you. Feel free to contact us if you have any questions or need further assistance. See you next time and don't miss to bookmark.