What Is The Difference Between Exergonic And Endergonic Reactions
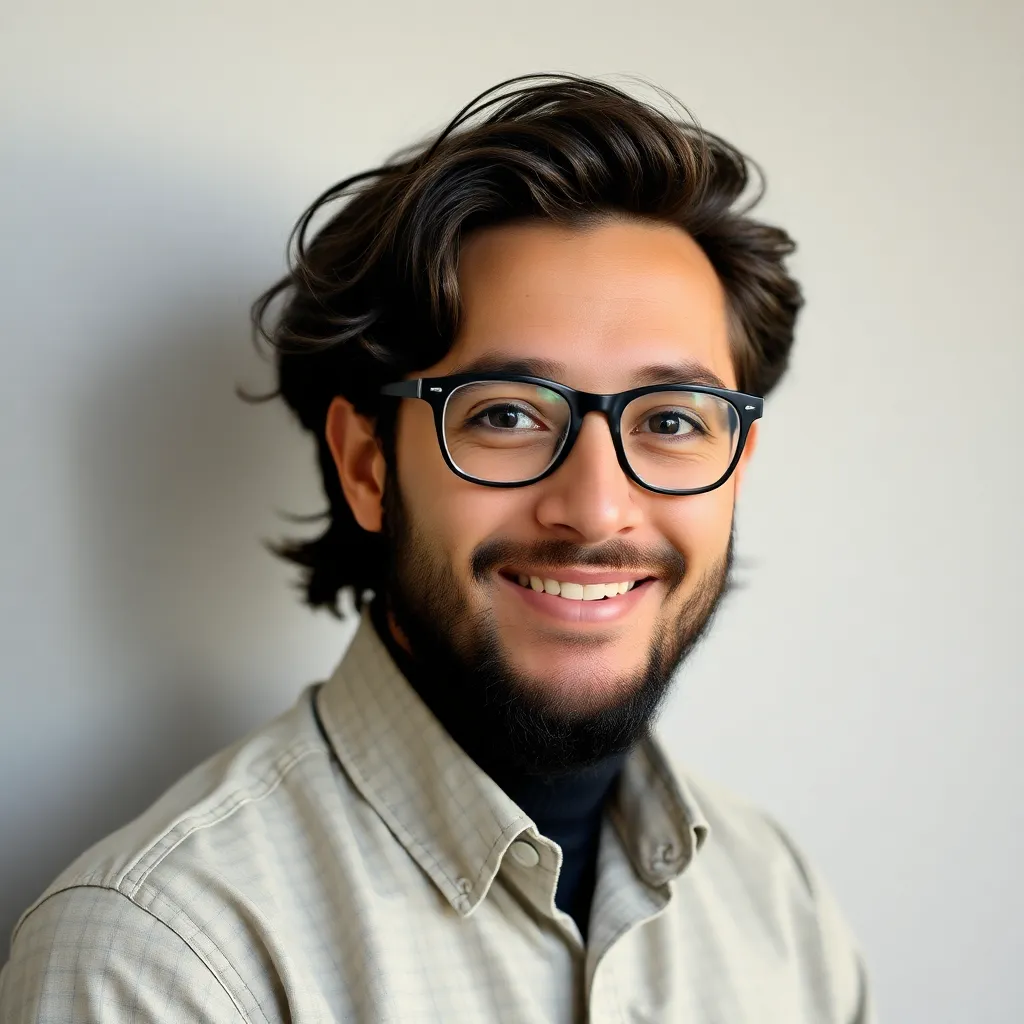
Muz Play
Apr 25, 2025 · 7 min read

Table of Contents
Exergonic vs. Endergonic Reactions: A Deep Dive into Energy Changes in Chemical Processes
Understanding the fundamental principles of energy transfer within chemical reactions is crucial for comprehending various biological and chemical processes. Two key concepts in this regard are exergonic reactions and endergonic reactions, which describe the direction of energy flow during a reaction. While seemingly complex, grasping the core differences between these two reaction types can significantly enhance your understanding of chemistry and biology. This article provides a comprehensive exploration of exergonic and endergonic reactions, detailing their characteristics, examples, and significance.
Defining Exergonic and Endergonic Reactions: Energy at the Core
At the heart of both exergonic and endergonic reactions lies the concept of Gibbs Free Energy (ΔG). Gibbs Free Energy represents the amount of energy available in a system to do useful work at a constant temperature and pressure. It's a crucial thermodynamic parameter that determines the spontaneity of a reaction.
-
Exergonic Reactions (ΔG < 0): These reactions release energy to their surroundings. The free energy of the products is lower than the free energy of the reactants. This means the reaction proceeds spontaneously, without requiring an external input of energy. Think of it as a downhill process – the system naturally moves towards a lower energy state.
-
Endergonic Reactions (ΔG > 0): These reactions require an input of energy from their surroundings to proceed. The free energy of the products is higher than the free energy of the reactants. They are non-spontaneous, meaning they won't occur naturally unless energy is supplied. This is akin to an uphill process – the system needs energy to reach a higher energy state.
The Role of Enthalpy (ΔH) and Entropy (ΔS)
While ΔG provides the overall picture, it's essential to understand the contributions of enthalpy (ΔH) and entropy (ΔS). The relationship is expressed by the following equation:
ΔG = ΔH - TΔS
Where:
-
ΔH represents the change in enthalpy, or heat content, of the system. A negative ΔH indicates an exothermic reaction (heat is released), while a positive ΔH indicates an endothermic reaction (heat is absorbed).
-
T represents the absolute temperature in Kelvin.
-
ΔS represents the change in entropy, or disorder, of the system. A positive ΔS indicates an increase in disorder (more randomness), while a negative ΔS indicates a decrease in disorder (more order).
This equation illustrates how both enthalpy and entropy contribute to the spontaneity of a reaction. A negative ΔG can be achieved even if ΔH is positive (endothermic) if the increase in entropy (positive ΔS) is sufficiently large and the temperature is high enough. Conversely, a reaction might be non-spontaneous (positive ΔG) even if it's exothermic (negative ΔH) if the decrease in entropy (negative ΔS) is significant.
Exergonic Reactions: Spontaneous Energy Release
Exergonic reactions, characterized by a negative ΔG, are fundamental to many biological processes. They drive numerous essential functions within living organisms, from metabolism to muscle contraction. The energy released is often utilized to power endergonic reactions, maintaining a delicate balance within the system.
Examples of Exergonic Reactions:
-
Cellular Respiration: The breakdown of glucose in the presence of oxygen to produce ATP (adenosine triphosphate), the primary energy currency of cells, is a highly exergonic process. This reaction releases a significant amount of energy, which is then harnessed to perform various cellular functions.
-
Hydrolysis of ATP: The breakdown of ATP into ADP (adenosine diphosphate) and inorganic phosphate (Pi) is another classic example. This reaction releases energy, which is often coupled with endergonic reactions to drive them forward.
-
Combustion Reactions: The burning of fuels like wood or propane is a highly exergonic reaction, releasing significant amounts of heat and light. These reactions involve the oxidation of fuel molecules, releasing energy stored in their chemical bonds.
-
Neutralization Reactions: The reaction between a strong acid and a strong base, resulting in the formation of water and a salt, is typically exergonic. The formation of stable water molecules leads to a release of energy.
Characteristics of Exergonic Reactions:
-
Spontaneous: They proceed without requiring an external energy input.
-
Negative ΔG: The change in Gibbs Free Energy is negative.
-
Energy Release: They release energy to their surroundings.
-
Product Stability: The products are generally more stable than the reactants.
Endergonic Reactions: Energy-Requiring Processes
Unlike exergonic reactions, endergonic reactions (ΔG > 0) require an input of energy to occur. This energy input often comes from coupling the endergonic reaction with an exergonic reaction, creating a coupled reaction system where the overall ΔG is negative.
Examples of Endergonic Reactions:
-
Photosynthesis: The process by which plants convert light energy into chemical energy in the form of glucose is a prime example. This reaction requires energy from sunlight to overcome the energy barrier and synthesize glucose from carbon dioxide and water.
-
Protein Synthesis: The building of proteins from amino acids is an endergonic process, requiring energy input from ATP hydrolysis to form peptide bonds.
-
Muscle Contraction: While the overall process involves both exergonic and endergonic steps, the actual contraction of muscle fibers requires energy input from ATP hydrolysis.
-
Active Transport: The movement of molecules across cell membranes against their concentration gradient (from low concentration to high concentration) requires energy input, often in the form of ATP hydrolysis.
Characteristics of Endergonic Reactions:
-
Non-Spontaneous: They do not occur naturally without an external energy input.
-
Positive ΔG: The change in Gibbs Free Energy is positive.
-
Energy Absorption: They absorb energy from their surroundings.
-
Product Instability: The products are generally less stable than the reactants.
Coupling Exergonic and Endergonic Reactions: The Power of Synergy
Living organisms rely heavily on coupling exergonic and endergonic reactions to drive essential metabolic processes. This coupling ensures that energy released from exergonic reactions is efficiently used to power endergonic reactions. The overall process remains favorable (negative ΔG) as long as the energy released by the exergonic reaction exceeds the energy required by the endergonic reaction.
Mechanisms of Coupling:
-
Direct Coupling: In some cases, the exergonic and endergonic reactions share a common intermediate. The energy released by one reaction is directly transferred to the other reaction through this shared intermediate. A classic example is the coupling of ATP hydrolysis with an endergonic reaction.
-
Indirect Coupling: In other cases, the exergonic reaction generates ATP, which then serves as an energy source for the endergonic reaction. This is a more common mechanism, allowing for efficient energy transfer across various cellular processes.
-
Redox Reactions: Many metabolic pathways rely on redox (reduction-oxidation) reactions. These reactions involve the transfer of electrons, with one molecule being oxidized (losing electrons) and another molecule being reduced (gaining electrons). Oxidation reactions are often exergonic, while reduction reactions can be endergonic.
The Significance of Exergonic and Endergonic Reactions in Biology and Beyond
The interplay between exergonic and endergonic reactions is fundamental to life itself. The efficient utilization of energy from exergonic reactions to power endergonic reactions is the foundation of metabolism, enabling organisms to perform a vast array of life-sustaining processes.
Biological Importance:
-
Metabolism: Exergonic and endergonic reactions are the building blocks of metabolic pathways, enabling organisms to synthesize essential molecules, break down food for energy, and maintain cellular homeostasis.
-
Energy Production: Exergonic reactions, such as cellular respiration, provide the energy required for various cellular processes, ensuring survival and growth.
-
Biosynthesis: Endergonic reactions, such as protein synthesis and DNA replication, are vital for building and maintaining the structural components of cells and organisms.
-
Homeostasis: The coupling of exergonic and endergonic reactions plays a crucial role in maintaining cellular balance and adapting to changing environmental conditions.
Industrial and Technological Applications:
The principles of exergonic and endergonic reactions also have numerous applications outside of biology. These principles are used in various industrial processes, including:
-
Chemical Synthesis: Understanding the energy changes involved in chemical reactions helps optimize reaction conditions for efficient synthesis of desired products.
-
Energy Production: Combustion reactions, a type of exergonic reaction, are the basis of many energy production technologies.
-
Materials Science: Controlling energy changes during material processing can lead to the development of novel materials with improved properties.
Conclusion: A Dynamic Energy Balance
The interplay between exergonic and endergonic reactions provides a comprehensive understanding of energy transformations in both biological and chemical systems. Exergonic reactions release energy, driving spontaneous processes, while endergonic reactions require energy input to proceed. The coupling of these two types of reactions is vital for life, enabling the efficient utilization of energy to power various essential processes. Understanding these principles opens the door to a deeper appreciation of the intricate energy dynamics that govern the world around us.
Latest Posts
Latest Posts
-
An Aqueous Solution Is One In Which Is The Solvent
Apr 25, 2025
-
Does Effective Nuclear Charge Decrease Down A Group
Apr 25, 2025
-
Who Used The Term Cell First
Apr 25, 2025
-
Antonie Van Leeuwenhoek Contribution To Cell Theory
Apr 25, 2025
-
Pressure Volume Work Is Also Called
Apr 25, 2025
Related Post
Thank you for visiting our website which covers about What Is The Difference Between Exergonic And Endergonic Reactions . We hope the information provided has been useful to you. Feel free to contact us if you have any questions or need further assistance. See you next time and don't miss to bookmark.