What Is The Monomer Of An Enzyme
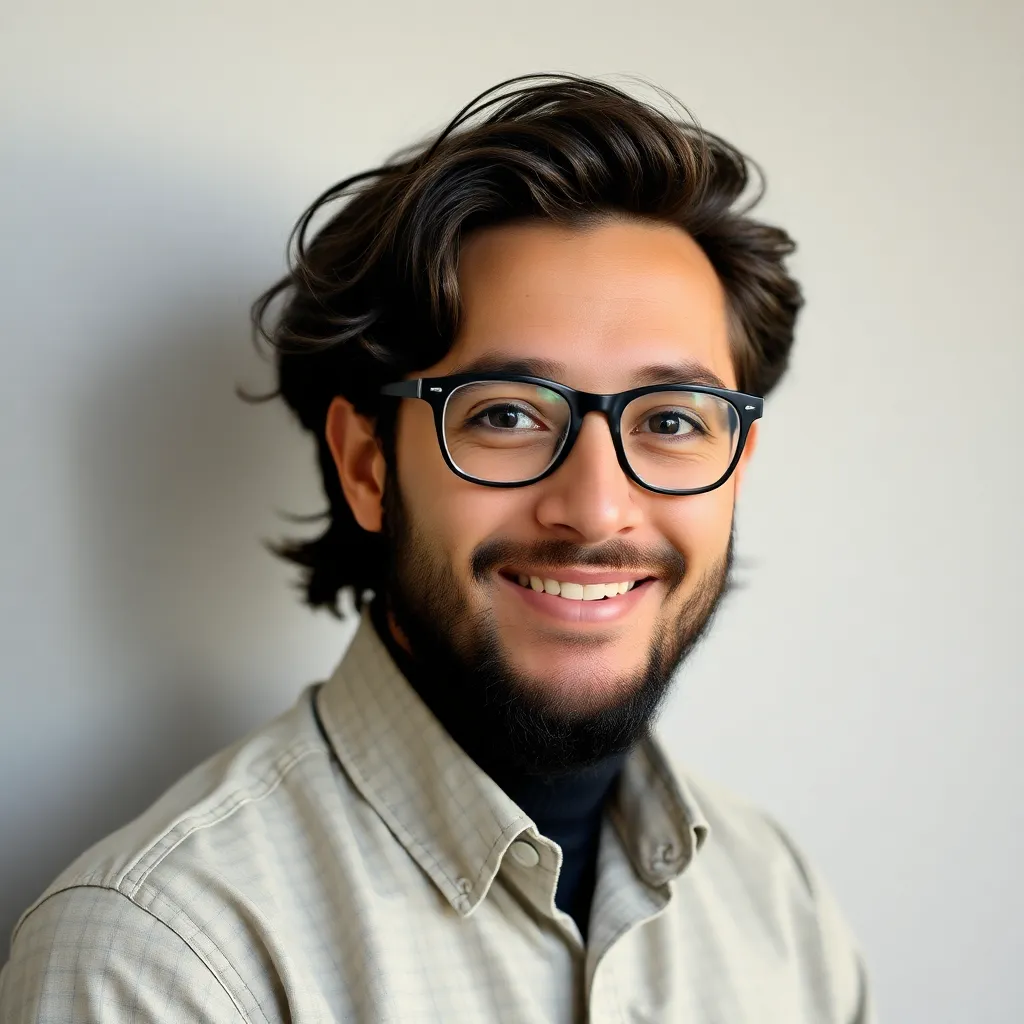
Muz Play
Apr 23, 2025 · 6 min read

Table of Contents
What is the Monomer of an Enzyme? Understanding the Building Blocks of Biological Catalysts
Enzymes, the biological catalysts that drive countless reactions within living organisms, are fascinating macromolecules with intricate structures and functions. Understanding their fundamental building blocks is crucial to comprehending their remarkable capabilities. This article delves deep into the question: What is the monomer of an enzyme? The answer, while seemingly straightforward, unveils a complex world of amino acids, peptide bonds, and the remarkable diversity of enzyme structures.
The Primary Building Blocks: Amino Acids
The monomer of an enzyme is the amino acid. These aren't just individual units; they are the fundamental building blocks of proteins, and enzymes themselves are proteins (with a few exceptions, such as ribozymes, which are RNA molecules). Amino acids are organic molecules characterized by a central carbon atom bonded to four groups:
- An amino group (-NH2): This is a basic group, meaning it can accept a proton (H+).
- A carboxyl group (-COOH): This is an acidic group, meaning it can donate a proton (H+).
- A hydrogen atom (-H): A simple hydrogen atom.
- A side chain (R-group): This is the variable group that distinguishes one amino acid from another. It's the R-group that dictates the amino acid's chemical properties, influencing its interactions with other amino acids and ultimately shaping the enzyme's overall structure and function.
There are 20 standard amino acids encoded by the genetic code, each with a unique R-group. These R-groups vary widely in their properties: some are nonpolar and hydrophobic (water-repelling), others are polar and hydrophilic (water-attracting), and some even carry a net positive or negative charge. This diversity is crucial for the formation of the complex three-dimensional structures essential for enzyme function.
Understanding the R-group's Significance
The diversity of R-groups significantly impacts the enzyme's properties. For instance:
- Hydrophobic R-groups: These often cluster together in the enzyme's interior, away from the aqueous environment of the cell. This hydrophobic interaction is a key driving force in protein folding.
- Hydrophilic R-groups: These tend to be located on the enzyme's surface, interacting with the surrounding water molecules.
- Charged R-groups: These can form ionic bonds with other charged groups, contributing significantly to the stability and specific interactions within the enzyme's structure.
The precise sequence of amino acids, known as the primary structure, dictates how the protein folds into its unique three-dimensional shape. This sequence is dictated by the genetic code, ensuring the accurate synthesis of the enzyme.
From Monomer to Polymer: Peptide Bonds and Protein Structure
Amino acids don't exist independently within an enzyme; they are linked together through peptide bonds. A peptide bond is formed through a dehydration reaction between the carboxyl group of one amino acid and the amino group of another. This reaction removes a water molecule, resulting in a covalent bond between the two amino acids.
The chain of amino acids connected by peptide bonds is called a polypeptide chain. This polypeptide chain then folds into a specific three-dimensional structure, which is essential for the enzyme's catalytic activity. This folding process involves several levels of structure:
- Primary structure: The linear sequence of amino acids in the polypeptide chain. This is determined directly by the gene encoding the enzyme.
- Secondary structure: Local folding patterns within the polypeptide chain, stabilized by hydrogen bonds between the amino and carboxyl groups of the peptide backbone. Common secondary structures include alpha-helices and beta-sheets.
- Tertiary structure: The overall three-dimensional arrangement of the polypeptide chain, stabilized by a variety of interactions, including hydrophobic interactions, hydrogen bonds, ionic bonds, and disulfide bridges. This structure defines the enzyme's active site, the region where the substrate binds and the catalytic reaction takes place.
- Quaternary structure: In some enzymes, multiple polypeptide chains (subunits) associate to form a functional enzyme. This interaction between subunits is also stabilized by various interactions, similar to those involved in tertiary structure.
The Active Site: Where the Magic Happens
The active site is a crucial region within the enzyme's tertiary structure. It's a three-dimensional pocket or cleft formed by specific amino acid residues. The active site is perfectly designed to bind to the enzyme's substrate (the molecule the enzyme acts upon). This highly specific binding is essential for the enzyme's catalytic activity. The precise arrangement of amino acid side chains within the active site facilitates the chemical reaction, lowering the activation energy required for the reaction to proceed.
Different enzymes have vastly different active sites, reflecting the diversity of their substrates and the reactions they catalyze. The shape and chemical properties of the active site are directly influenced by the primary structure and subsequent folding of the polypeptide chain – all originating from the sequence of amino acid monomers.
Enzyme Classification and Diversity
Enzymes are classified into six main classes based on the type of reaction they catalyze:
- Oxidoreductases: Catalyze oxidation-reduction reactions (transfer of electrons).
- Transferases: Catalyze the transfer of functional groups between molecules.
- Hydrolases: Catalyze hydrolysis reactions (breaking bonds using water).
- Lyases: Catalyze the addition or removal of groups to form double bonds.
- Isomerases: Catalyze the rearrangement of atoms within a molecule.
- Ligases: Catalyze the joining of two molecules using ATP (adenosine triphosphate).
Each of these enzyme classes contains a vast number of different enzymes, each with a unique amino acid sequence and specific three-dimensional structure tailored to its particular function. This diversity is a testament to the power of the 20 amino acid monomers and the incredibly complex ways they can assemble to create such a wide range of biological catalysts.
Beyond the 20 Standard Amino Acids: Post-Translational Modifications
While the 20 standard amino acids are the primary building blocks of enzymes, post-translational modifications can further diversify the properties of the protein. These modifications occur after the polypeptide chain is synthesized and can significantly impact the enzyme's activity, stability, and interactions with other molecules. Examples of post-translational modifications include:
- Phosphorylation: The addition of a phosphate group, often altering the enzyme's activity.
- Glycosylation: The addition of carbohydrate groups, affecting the enzyme's stability and interactions.
- Acetylation: The addition of an acetyl group, influencing protein stability and function.
These modifications highlight the dynamic nature of enzymes and the complexity of their regulation within the cell. They demonstrate that the simple amino acid monomer is just the starting point in a complex cascade of events that ultimately leads to a functional, highly specific, and exquisitely regulated biological catalyst.
Conclusion: The Intricate World of Enzyme Monomers
In essence, the monomer of an enzyme is the amino acid. However, this simple answer belies the incredible complexity of enzyme structure and function. The 20 standard amino acids, linked together by peptide bonds, fold into intricate three-dimensional structures that define the enzyme's active site and catalytic properties. Post-translational modifications add further layers of complexity, highlighting the dynamic nature of these biological workhorses. Understanding the structure and function of enzymes, starting with their amino acid monomers, is fundamental to comprehending the intricate machinery of life. The precise arrangement of these monomers determines the catalytic properties, substrate specificity, and regulation of these essential molecules, making them indispensable for nearly every biological process imaginable.
Latest Posts
Latest Posts
-
Which Set Of Regions In The Abdominopelvic Cavity Is Medial
Apr 23, 2025
-
Is Nitrogen A Cation Or Anion
Apr 23, 2025
-
Using Wedges And Dashes In Skeletal Structures
Apr 23, 2025
-
What Determines The Properties Of An Element
Apr 23, 2025
-
A Chromosome Has An Inversion Which Describes A Pericentric Inversion
Apr 23, 2025
Related Post
Thank you for visiting our website which covers about What Is The Monomer Of An Enzyme . We hope the information provided has been useful to you. Feel free to contact us if you have any questions or need further assistance. See you next time and don't miss to bookmark.