What Is The Reaction That Links Two Monosaccharides Together
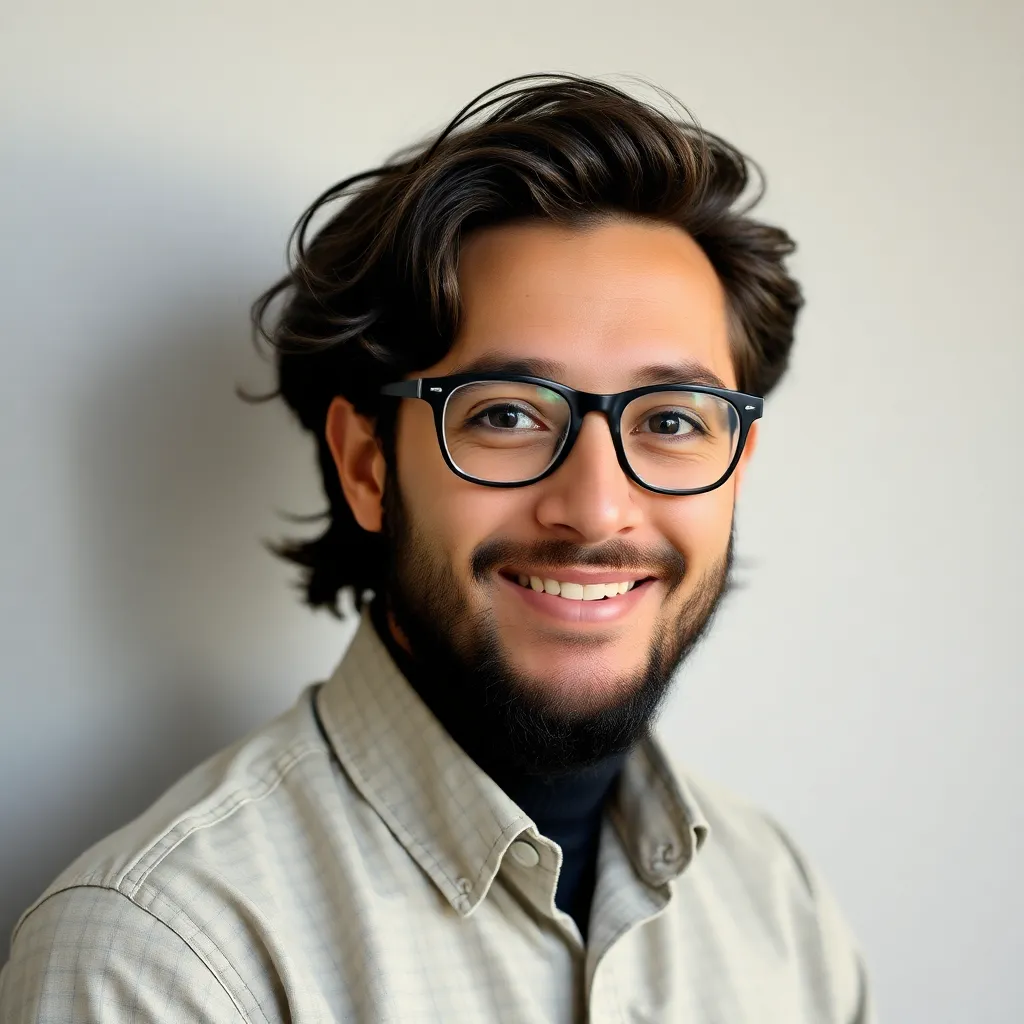
Muz Play
May 09, 2025 · 6 min read
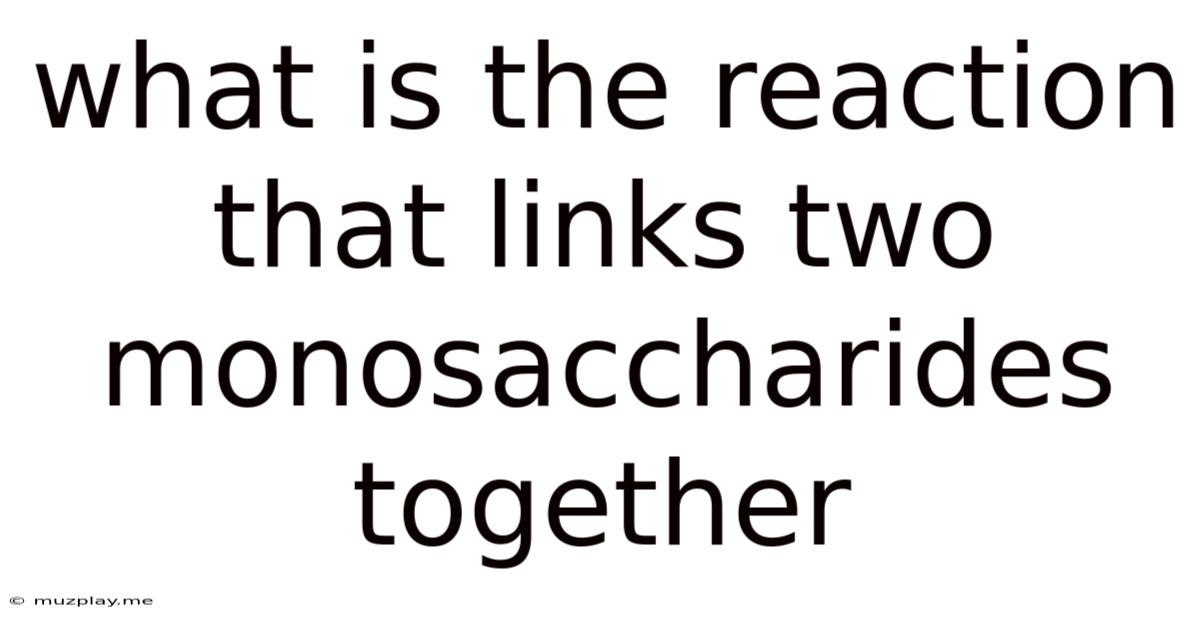
Table of Contents
What is the Reaction that Links Two Monosaccharides Together?
Glycosidic bonds are the cornerstone of carbohydrate chemistry, linking monosaccharides to form disaccharides, oligosaccharides, and the vast, complex polymers known as polysaccharides. Understanding the formation of these bonds is crucial to grasping the structure and function of carbohydrates, which play vital roles in energy storage, structural support, and cellular communication. This article delves deep into the reaction that links two monosaccharides together, exploring the mechanism, types of bonds, and the implications of glycosidic linkage for the properties of the resulting carbohydrate.
The Glycosidic Bond: A Covalent Link
The reaction that joins two monosaccharides is a dehydration synthesis, also known as a condensation reaction. This process involves the removal of a water molecule (H₂O) to form a new covalent bond between the two monosaccharide units. Specifically, a hydroxyl group (-OH) from one monosaccharide and a hydrogen atom (-H) from the hydroxyl group of the other monosaccharide are released as water, leaving behind an oxygen atom that bridges the two monosaccharide rings, forming the glycosidic bond.
The Mechanism of Glycosidic Bond Formation
The formation of a glycosidic bond is catalyzed by enzymes called glycosyltransferases. These enzymes play a critical role in orchestrating the precise linking of monosaccharides during various metabolic pathways. The detailed mechanism involves several steps:
-
Activation of the monosaccharide: One monosaccharide acts as a donor and needs to be activated. This often involves the attachment of a nucleotide diphosphate (such as UDP or GDP) to the monosaccharide, forming a high-energy intermediate. This activation increases the reactivity of the monosaccharide's anomeric carbon.
-
Nucleophilic attack: The activated monosaccharide's anomeric carbon (the carbon atom that is part of the carbonyl group in the open-chain form of the monosaccharide) acts as an electrophile. The hydroxyl group of the acceptor monosaccharide acts as a nucleophile, attacking the anomeric carbon.
-
Glycosidic bond formation: The nucleophilic attack results in the formation of a new covalent bond between the anomeric carbon of the donor monosaccharide and the hydroxyl group of the acceptor monosaccharide. A water molecule is released in the process.
-
Release of the product: The resulting disaccharide is released from the enzyme, completing the reaction.
Types of Glycosidic Bonds
Glycosidic bonds are classified based on several factors, and understanding these classifications is essential for comprehending the diversity and functionality of carbohydrates:
Based on the Anomeric Carbon: α or β
The anomeric carbon is the carbon atom that was part of the carbonyl group in the open-chain form of the monosaccharide. After cyclization, it becomes a chiral center. The glycosidic bond can form either above or below the plane of the ring, leading to two distinct configurations:
-
α (alpha) linkage: The glycosidic bond is formed below the plane of the ring (if the -OH group on the anomeric carbon is pointing down in the Haworth projection).
-
β (beta) linkage: The glycosidic bond is formed above the plane of the ring (if the -OH group on the anomeric carbon is pointing up in the Haworth projection).
This seemingly small difference in stereochemistry has profound effects on the properties of the resulting polysaccharide. For example, starch (amylose and amylopectin) contains α-1,4-glycosidic linkages, making it readily digestible by humans, while cellulose contains β-1,4-glycosidic linkages, which are indigestible by humans due to the lack of the necessary enzymes to break them down.
Based on the Carbon Atoms Involved: 1,2; 1,4; 1,6 etc.
The numbers in the designation of a glycosidic bond indicate the carbon atoms involved in the linkage. For example:
-
α-1,4-glycosidic bond: The bond is formed between carbon 1 of the first monosaccharide and carbon 4 of the second monosaccharide, with an α-configuration at carbon 1. This is the type of bond found in amylose, a component of starch.
-
β-1,4-glycosidic bond: The bond is formed between carbon 1 of the first monosaccharide and carbon 4 of the second monosaccharide, with a β-configuration at carbon 1. This is the type of bond found in cellulose.
-
α-1,6-glycosidic bond: The bond is formed between carbon 1 of one monosaccharide and carbon 6 of another. This type of branching is commonly found in amylopectin, a branched form of starch.
The variations in the carbons involved in the glycosidic linkage contribute to the structural diversity and functionality of polysaccharides. Branching, introduced by linkages such as 1,6-glycosidic bonds, significantly impacts the physical properties of the polysaccharide, influencing factors like solubility and digestibility.
Examples of Glycosidic Bonds in Important Carbohydrates
Understanding the significance of glycosidic bonds requires examining specific examples of how they contribute to the structure and function of various carbohydrates:
Sucrose (Table Sugar): α-1,2-glycosidic bond
Sucrose, the common table sugar, is a disaccharide formed by the linkage of glucose and fructose via an α-1,2-glycosidic bond. This bond connects the anomeric carbon of glucose to the anomeric carbon of fructose.
Lactose (Milk Sugar): β-1,4-glycosidic bond
Lactose, the sugar found in milk, is a disaccharide composed of galactose and glucose linked by a β-1,4-glycosidic bond. The bond connects the anomeric carbon of galactose to carbon 4 of glucose.
Maltose (Malt Sugar): α-1,4-glycosidic bond
Maltose, a product of starch hydrolysis, is a disaccharide formed from two glucose molecules linked by an α-1,4-glycosidic bond.
Starch (Amylose and Amylopectin): α-1,4 and α-1,6-glycosidic bonds
Starch, a major energy storage polysaccharide in plants, consists of amylose and amylopectin. Amylose is a linear polymer of glucose units linked by α-1,4-glycosidic bonds. Amylopectin, a branched polymer, also has α-1,4-glycosidic bonds in its linear chains, but also features α-1,6-glycosidic bonds at branch points.
Cellulose: β-1,4-glycosidic bonds
Cellulose, the primary structural component of plant cell walls, is a linear polymer of glucose units linked by β-1,4-glycosidic bonds. The β-linkage leads to a straight chain structure, allowing for extensive hydrogen bonding between adjacent cellulose molecules, resulting in a strong and rigid structure.
Glycogen: α-1,4 and α-1,6-glycosidic bonds
Glycogen, the main energy storage polysaccharide in animals, is similar in structure to amylopectin, containing both α-1,4 and α-1,6-glycosidic bonds. However, glycogen is more highly branched than amylopectin, allowing for rapid mobilization of glucose molecules when needed.
The Significance of Glycosidic Bond Variations
The variations in glycosidic bond types are not merely structural details; they have profound implications for the biological functions of carbohydrates:
-
Digestibility: The type of glycosidic bond dictates whether a carbohydrate can be digested by a particular organism. Humans can digest α-glycosidic bonds but lack the enzymes to break down β-glycosidic bonds, explaining why we can digest starch but not cellulose.
-
Solubility: The conformation of the polysaccharide determined by the glycosidic linkage affects its solubility in water. Branched polysaccharides tend to be more soluble than linear ones.
-
Reactivity: The type and location of glycosidic bonds influence the reactivity of the carbohydrate molecule, determining its interactions with other biomolecules.
-
Biological function: The unique properties conferred by different types of glycosidic bonds are crucial for the diverse biological roles of carbohydrates, ranging from energy storage and structural support to cell recognition and signaling.
Conclusion
The reaction that links two monosaccharides together, a dehydration synthesis forming a glycosidic bond, is a fundamental process in carbohydrate chemistry and biology. The specific type of glycosidic bond formed – α or β, and the carbon atoms involved – significantly impacts the properties and biological roles of the resulting carbohydrate. The remarkable diversity of carbohydrate structures arises from the subtle yet impactful variations in glycosidic linkages, highlighting the elegance and complexity of these essential biomolecules. Understanding these linkages is crucial for comprehending various biological processes and developing applications in fields like medicine and biotechnology.
Latest Posts
Latest Posts
-
Having Two Different Genes For A Trait Sometimes Called Hybrid
May 09, 2025
-
Alkaline Earth Metal In Period 6
May 09, 2025
-
How Did Matthias Schleiden Contribute To The Cell Theory
May 09, 2025
-
Notes On Newtons Laws Of Motion
May 09, 2025
-
How To Find Current Through Each Resistor
May 09, 2025
Related Post
Thank you for visiting our website which covers about What Is The Reaction That Links Two Monosaccharides Together . We hope the information provided has been useful to you. Feel free to contact us if you have any questions or need further assistance. See you next time and don't miss to bookmark.