What Is The Sliding Filament Model Of Muscle Contraction
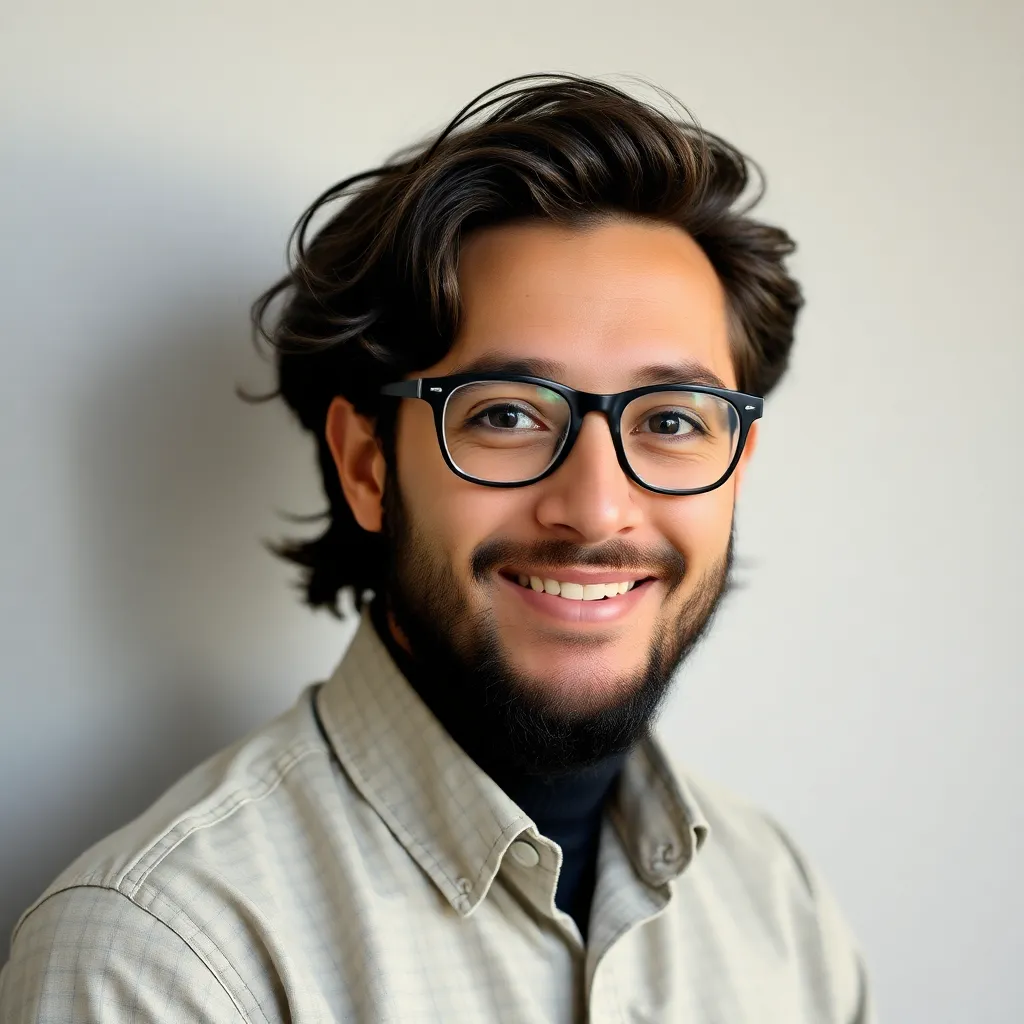
Muz Play
Apr 17, 2025 · 7 min read

Table of Contents
What is the Sliding Filament Model of Muscle Contraction?
The sliding filament theory, a cornerstone of muscle physiology, elegantly explains how muscles generate force and movement. Instead of muscle fibers shortening, as once believed, this model proposes that muscle contraction results from the sliding of filaments past one another within the muscle fiber's sarcomeres. This intricate process, fueled by ATP (adenosine triphosphate), allows muscles to perform a vast range of actions, from subtle finger movements to powerful leg strides. This article will delve deep into the sliding filament theory, exploring its mechanisms, key players, and significance in various muscle types.
The Structure of Skeletal Muscle: Setting the Stage
To understand the sliding filament model, it's crucial to first grasp the structural organization of skeletal muscle. Skeletal muscle is composed of bundles of muscle fibers, which themselves are made up of numerous myofibrils. These myofibrils are the fundamental units responsible for muscle contraction. The myofibrils exhibit a repeating pattern of light and dark bands under a microscope, giving them a striated appearance. This striated pattern is due to the precise arrangement of two key protein filaments:
Myosin: The Thick Filament
Myosin filaments are thick filaments composed of hundreds of myosin molecules. Each myosin molecule resembles a golf club with a head and a tail. The myosin head possesses ATPase activity, an enzyme that hydrolyzes ATP, releasing energy necessary for muscle contraction. The myosin heads project outwards from the filament, creating cross-bridges that interact with the thin filaments.
Actin: The Thin Filament
Actin filaments are thin filaments predominantly composed of actin molecules, arranged in a helical structure. Two other important proteins are associated with actin:
- Tropomyosin: This long, fibrous protein winds around the actin filament, covering the myosin-binding sites on actin in a relaxed muscle.
- Troponin: This protein complex is bound to both tropomyosin and actin. It plays a crucial role in regulating muscle contraction by controlling tropomyosin's position. Troponin has three subunits: troponin I (inhibits actin-myosin interaction), troponin T (binds to tropomyosin), and troponin C (binds to calcium ions).
Sarcomeres: The Functional Units
Myosin and actin filaments are organized within highly structured units called sarcomeres. Each sarcomere is bounded by Z-lines, and it's the basic contractile unit of a muscle fiber. Within the sarcomere, the arrangement of myosin and actin filaments creates the characteristic striated pattern. The A-band (anisotropic band) contains both myosin and actin filaments, while the I-band (isotropic band) contains only actin filaments. The H-zone, situated within the A-band, contains only myosin filaments.
The Sliding Filament Mechanism: A Step-by-Step Explanation
The sliding filament model posits that muscle contraction occurs through the relative sliding of actin and myosin filaments over each other. This sliding shortens the sarcomere, leading to the overall shortening of the muscle fiber and subsequent muscle contraction. Here’s a detailed breakdown of the process:
-
Nerve Impulse and Calcium Release: Muscle contraction is initiated by a nerve impulse arriving at the neuromuscular junction. This impulse triggers the release of acetylcholine, a neurotransmitter, which depolarizes the muscle fiber membrane. This depolarization then spreads into the T-tubules (transverse tubules), invaginations of the muscle fiber membrane. The T-tubules are closely associated with the sarcoplasmic reticulum (SR), a specialized intracellular calcium store. Depolarization of the T-tubules triggers the release of calcium ions (Ca²⁺) from the SR into the sarcoplasm (muscle fiber cytoplasm).
-
Calcium Binding to Troponin: The increased cytosolic calcium concentration binds to troponin C. This binding causes a conformational change in troponin, which in turn moves tropomyosin away from the myosin-binding sites on the actin filament. This uncovers the binding sites, allowing the myosin heads to interact with actin.
-
Cross-bridge Formation and Power Stroke: The myosin heads, now energized by ATP hydrolysis, bind to the exposed myosin-binding sites on actin, forming cross-bridges. The binding of the myosin head to actin triggers the power stroke, a conformational change in the myosin head that pulls the actin filament towards the center of the sarcomere. This power stroke generates the force of muscle contraction. ADP and inorganic phosphate (Pi) are released during this step.
-
Cross-bridge Detachment and ATP Binding: Following the power stroke, a new ATP molecule binds to the myosin head. This binding causes the myosin head to detach from the actin filament.
-
ATP Hydrolysis and Myosin Head Re-cocking: The ATP bound to the myosin head is hydrolyzed, providing energy for the myosin head to return to its original high-energy conformation, ready to bind to another actin-binding site and repeat the cycle.
-
Cycle Repetition: This cycle of cross-bridge formation, power stroke, detachment, and re-cocking repeats continuously as long as calcium ions are present in the sarcoplasm and ATP is available. The continuous cycling of myosin heads pulling on the actin filaments leads to the sliding of the filaments and the shortening of the sarcomere.
-
Muscle Relaxation: When the nerve impulse ceases, calcium ions are actively pumped back into the SR by calcium ATPase pumps. This reduction in cytosolic calcium concentration causes troponin C to release calcium, allowing tropomyosin to cover the myosin-binding sites on actin again. Cross-bridge cycling stops, and the muscle relaxes.
The Role of ATP in Muscle Contraction
ATP plays a pivotal role in muscle contraction, fulfilling three crucial functions:
-
Energizing the Myosin Head: ATP hydrolysis provides the energy needed for the myosin head to undergo conformational changes, allowing it to bind to actin, perform the power stroke, and detach.
-
Cross-bridge Detachment: ATP binding is necessary for the detachment of the myosin head from actin, preventing the muscle from remaining locked in a contracted state.
-
Calcium Pump Function: ATP is required for the active transport of calcium ions back into the SR, a crucial step in muscle relaxation. Without sufficient ATP, muscles would remain contracted, leading to rigor mortis.
Different Types of Muscle Contraction
The sliding filament model applies to all three types of muscle tissue – skeletal, smooth, and cardiac – although the details of regulation and structure vary:
Skeletal Muscle Contraction:
Skeletal muscle contractions are characterized by rapid and forceful contractions, precisely controlled by the nervous system. The sliding filament mechanism operates as described above, with calcium release triggering cross-bridge cycling.
Smooth Muscle Contraction:
Smooth muscle contractions are slower and more sustained than skeletal muscle contractions. Calcium entry from the extracellular fluid is a key trigger, and the regulatory mechanisms involving calmodulin and myosin light chain kinase differ from the troponin-tropomyosin system in skeletal muscle.
Cardiac Muscle Contraction:
Cardiac muscle contractions are rhythmic and involuntary, controlled by the intrinsic conduction system of the heart. The sliding filament mechanism is also at play, but calcium-induced calcium release from the SR plays a significant role in generating the contractile force.
Clinical Significance and Related Disorders
Understanding the sliding filament model is crucial for comprehending various muscle-related disorders. Disruptions in any aspect of the process can lead to muscle weakness or dysfunction. Examples include:
-
Muscle Dystrophy: A group of genetic disorders characterized by progressive muscle weakness and degeneration, often involving defects in proteins crucial for muscle structure and function.
-
Myasthenia Gravis: An autoimmune disorder affecting neuromuscular transmission, leading to muscle weakness and fatigue.
-
Muscular Dystrophy: Inherited diseases that cause progressive muscle weakness and degeneration due to genetic defects affecting muscle proteins.
-
Rigor Mortis: The stiffening of muscles after death due to the depletion of ATP, leading to persistent cross-bridge attachment.
Conclusion: A Dynamic and Essential Process
The sliding filament model provides a comprehensive framework for understanding muscle contraction. This elegantly simple yet powerfully intricate mechanism, driven by ATP hydrolysis and regulated by calcium ions, underpins movement and a vast array of bodily functions. Its importance is underscored by the various muscle-related disorders that arise from disruptions in this fundamental process. Further research continues to refine our understanding of the nuanced details of muscle contraction, promising advancements in the diagnosis and treatment of muscle-related conditions. The sliding filament model remains a central pillar of muscle physiology, highlighting the beauty and complexity of biological systems.
Latest Posts
Latest Posts
-
The Y 5 Molar Morphology Is Present In
Apr 19, 2025
-
Another Name For The Evolutionary Force Called Gene Flow Is
Apr 19, 2025
-
What Goes In A Trial Balance
Apr 19, 2025
-
How Many Protons And Neutrons Does Boron Have
Apr 19, 2025
-
In The Process Of Science Which Of These Is Tested
Apr 19, 2025
Related Post
Thank you for visiting our website which covers about What Is The Sliding Filament Model Of Muscle Contraction . We hope the information provided has been useful to you. Feel free to contact us if you have any questions or need further assistance. See you next time and don't miss to bookmark.