What Property Do All Muscle Tissues Have In Common
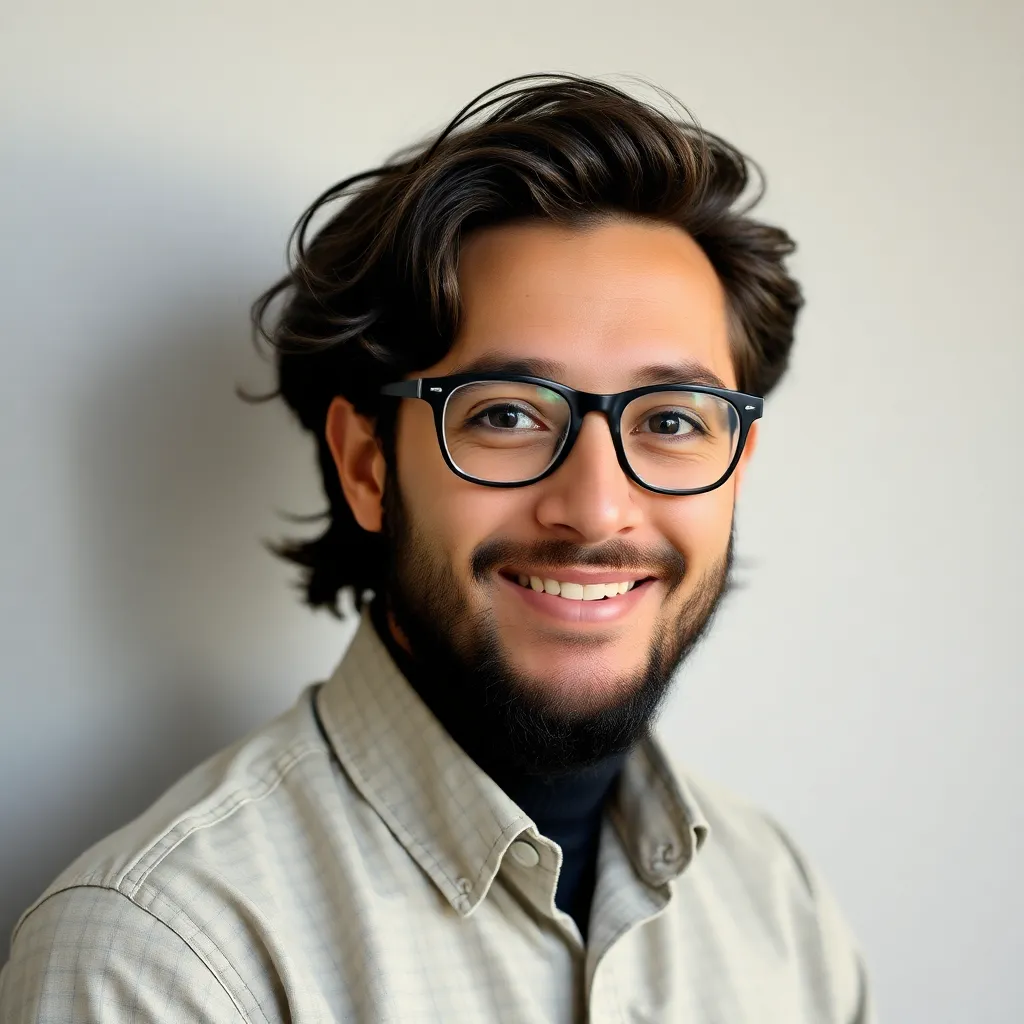
Muz Play
Apr 19, 2025 · 6 min read

Table of Contents
What Property Do All Muscle Tissues Have in Common? The Power of Contractility
The human body is a marvel of engineering, a complex symphony of interacting systems working in perfect harmony. At the heart of much of this movement and function lies muscle tissue, responsible for everything from the beating of our hearts to the subtle twitch of a finger. While different types of muscle tissue exist, exhibiting unique characteristics tailored to their specific roles, they all share one fundamental property: contractility. This essay will delve deep into the nature of contractility, exploring its underlying mechanisms and how this shared property allows for the diverse functionalities of skeletal, smooth, and cardiac muscle.
Understanding Contractility: The Defining Feature of Muscle Tissue
Contractility is the ability of muscle tissue to shorten forcefully. This seemingly simple definition belies a complex interplay of cellular structures and biochemical processes. It's not merely about getting shorter; it's about generating tension, the force exerted by the muscle fibers. This tension can lead to a variety of actions, depending on the type of muscle and its context. It's this ability to generate tension that allows muscles to move bones, propel blood through the circulatory system, and control the diameter of blood vessels, amongst many other vital functions.
The Molecular Machinery of Contraction: Actin and Myosin
At the microscopic level, contractility is driven by the interaction between two key proteins: actin and myosin. These proteins are arranged in highly organized structures within muscle cells, forming the sarcomeres, the basic functional units of muscle contraction. Myosin filaments, possessing "heads" that can bind to actin, are interwoven with actin filaments.
The process of contraction involves a complex series of steps:
-
Calcium Ion Release: The contraction cycle begins with the release of calcium ions (Ca²⁺) into the cytoplasm of the muscle cell. This calcium release is triggered by nerve impulses or other stimuli, depending on the type of muscle.
-
Cross-Bridge Formation: The calcium ions bind to a protein called troponin, causing a conformational change that exposes the myosin-binding sites on the actin filaments. Myosin heads then attach to these sites, forming cross-bridges.
-
Power Stroke: Once attached, the myosin heads undergo a conformational change, pivoting and pulling the actin filaments towards the center of the sarcomere. This is the power stroke, generating the force of muscle contraction.
-
Cross-Bridge Detachment: After the power stroke, the myosin heads detach from the actin filaments with the help of ATP (adenosine triphosphate), the body's energy currency.
-
ATP Hydrolysis and Reset: ATP is then hydrolyzed (broken down), providing the energy for the myosin heads to return to their original position, ready for another cycle.
This cycle of cross-bridge formation, power stroke, detachment, and reset repeats numerous times, resulting in the overall shortening of the muscle fiber. The speed and extent of contraction are regulated by factors such as the frequency of nerve impulses, the concentration of calcium ions, and the availability of ATP.
The Diverse Manifestations of Contractility: Exploring Muscle Tissue Types
While all muscle tissues share the fundamental property of contractility, the specific characteristics and functions of different muscle types are highly diverse. This diversity arises from variations in their structure, organization, and control mechanisms. Let's examine the three main types:
1. Skeletal Muscle: Voluntary Movement and Posture
Skeletal muscle, also known as striated muscle, is responsible for voluntary movements. It's attached to bones via tendons and is responsible for locomotion, facial expressions, posture maintenance, and a vast array of other voluntary actions. Its characteristic striated appearance comes from the highly organized arrangement of actin and myosin filaments into sarcomeres. Skeletal muscle fibers are multinucleated and relatively large, allowing for powerful contractions. Their contractions are rapid, forceful, and easily fatigued. Control is exerted by the somatic nervous system, allowing for conscious and precise control of movement. The speed of contraction is highly variable, depending on the specific muscle and the intensity of stimulation.
2. Smooth Muscle: Involuntary Actions in Internal Organs
Smooth muscle, unlike skeletal muscle, lacks the striated appearance. Its actin and myosin filaments are not arranged in the same orderly manner, resulting in a less organized structure. Smooth muscle is found in the walls of internal organs, such as the digestive tract, blood vessels, and urinary bladder. Its contractions are slow, sustained, and involuntary, meaning they are not under conscious control. The autonomic nervous system regulates smooth muscle contractions, ensuring the smooth and coordinated function of internal organs. Smooth muscle exhibits remarkable plasticity, meaning it can maintain tension for extended periods without fatigue. This is crucial for functions such as maintaining blood pressure and regulating digestion.
3. Cardiac Muscle: The Engine of the Heart
Cardiac muscle is found exclusively in the heart. Like skeletal muscle, it displays a striated appearance due to the organized arrangement of sarcomeres. However, it differs significantly in its structure and function. Cardiac muscle cells are interconnected via specialized junctions called intercalated discs, allowing for the rapid and coordinated spread of electrical signals throughout the heart. This ensures a synchronized contraction of the heart chambers, essential for efficient blood pumping. Cardiac muscle contractions are involuntary and rhythmic, controlled by the intrinsic conduction system of the heart, supplemented by regulation from the autonomic nervous system. Cardiac muscle exhibits a unique property of automaticity, meaning it can generate its own electrical impulses, initiating its own contractions. Its contractions are strong, rhythmic, and resistant to fatigue, ensuring the continuous pumping of blood throughout life.
Contractility Beyond the Basics: Factors Influencing Muscle Performance
The ability of muscle tissue to contract is influenced by several key factors:
-
Neural Stimulation: The frequency and intensity of nerve impulses play a crucial role in determining the strength and duration of muscle contractions.
-
Hormonal Influence: Hormones such as adrenaline can significantly enhance muscle contractility, increasing both the strength and speed of contractions.
-
Nutrient Availability: Adequate supplies of ATP, derived from glucose and other energy substrates, are essential for sustained muscle contraction. A lack of nutrients can lead to muscle fatigue.
-
Oxygen Supply: Muscle contraction requires a considerable supply of oxygen to support the metabolic processes involved in ATP production. Insufficient oxygen supply can lead to anaerobic metabolism and the production of lactic acid, resulting in muscle fatigue.
-
Muscle Fiber Type: Different muscle fiber types (e.g., slow-twitch and fast-twitch) have varying capacities for contraction speed, power, and fatigue resistance.
-
Temperature: Muscle contractility is temperature-dependent, with optimal performance occurring within a specific temperature range.
Clinical Significance of Contractility: Diseases and Disorders
Impaired contractility can manifest in a variety of muscle-related diseases and disorders, including:
-
Muscular Dystrophy: A group of inherited diseases characterized by progressive muscle weakness and degeneration.
-
Myasthenia Gravis: An autoimmune disorder affecting the neuromuscular junction, leading to muscle weakness and fatigue.
-
Heart Failure: Impaired contractility of the heart muscle can lead to heart failure, a serious condition affecting the heart's ability to pump blood effectively.
-
Smooth Muscle Dysfunction: Problems with smooth muscle contractility can affect various bodily functions, including digestion, blood pressure regulation, and urinary function.
Conclusion: The Unifying Force of Contractility
In conclusion, contractility is the defining property of all muscle tissues. While the specific manifestations of this property vary considerably among skeletal, smooth, and cardiac muscle, the underlying principle—the ability to generate force through the interaction of actin and myosin—remains constant. Understanding the mechanisms of contractility and the factors that influence it is fundamental to appreciating the remarkable diversity and functionality of muscle tissue, its crucial role in maintaining health, and the implications of its dysfunction in various diseases. Future research in this field holds promise for developing new therapeutic strategies for treating a wide range of muscle-related disorders.
Latest Posts
Latest Posts
-
What Are The Most Active Nonmetals
Apr 19, 2025
-
Identify The Axial And Equatorial Atoms In The Three Dimensional Structure
Apr 19, 2025
-
How To Find Electrons In An Isotope
Apr 19, 2025
-
Transcription Begins Near A Site In The Dna Called The
Apr 19, 2025
-
Conventional Current Flows From Positive To Negative
Apr 19, 2025
Related Post
Thank you for visiting our website which covers about What Property Do All Muscle Tissues Have In Common . We hope the information provided has been useful to you. Feel free to contact us if you have any questions or need further assistance. See you next time and don't miss to bookmark.