When Matter Is Converted In A Nuclear Reaction
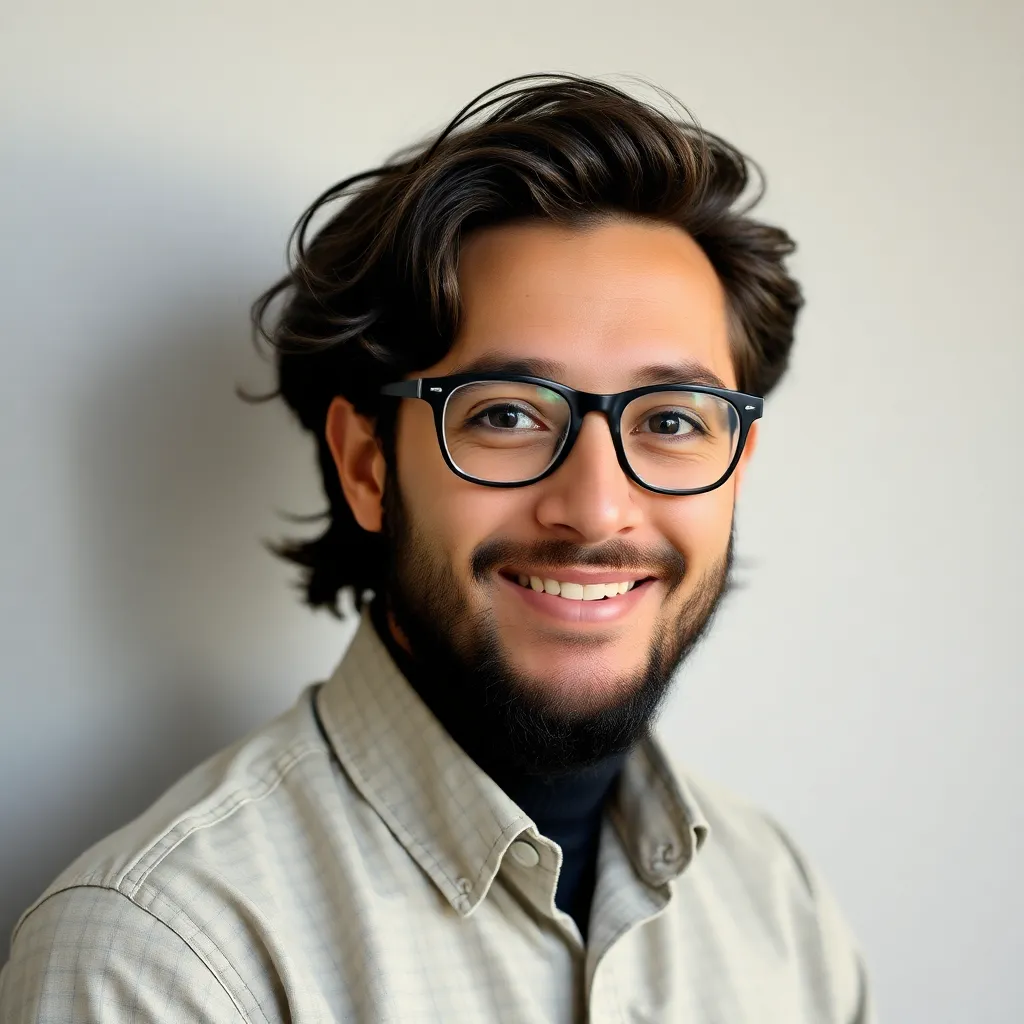
Muz Play
Apr 18, 2025 · 7 min read

Table of Contents
When Matter is Converted in a Nuclear Reaction: Unveiling the Secrets of Mass-Energy Equivalence
Nuclear reactions, unlike chemical reactions, involve transformations at the heart of the atom – its nucleus. These reactions can unleash tremendous amounts of energy, a phenomenon deeply connected to the conversion of matter into energy, a concept famously encapsulated by Einstein's equation, E=mc². Understanding this conversion requires delving into the fundamental principles of nuclear physics and the intricacies of nuclear forces.
The Nucleus: A Realm of Strong Forces
The atom's nucleus, a tiny but incredibly dense region, comprises protons and neutrons, collectively known as nucleons. These particles are held together by the strong nuclear force, a fundamental force far stronger than the electromagnetic force that repels positively charged protons. However, the strong force has a very short range, effective only over distances comparable to the size of the nucleus. This limited range explains why nuclei don't collapse under their own gravitational pull, and why the stability of a nucleus depends critically on the delicate balance between the strong force and the electromagnetic repulsion.
Isotopes and Nuclear Stability
Atoms of the same element can have different numbers of neutrons, forming isotopes. Some isotopes are stable, meaning their nuclei remain intact indefinitely. Others are unstable, or radioactive, undergoing spontaneous transformations to achieve a more stable configuration. This transformation often involves the emission of particles or energy, a process central to nuclear reactions and matter-energy conversion.
Nuclear Reactions: Fission and Fusion
Nuclear reactions broadly fall into two categories: fission and fusion.
Fission: Splitting the Atom
Nuclear fission involves the splitting of a heavy atomic nucleus into two or more lighter nuclei. This process is typically initiated by bombarding the heavy nucleus with neutrons. The resulting lighter nuclei possess a smaller combined mass than the original nucleus. This "missing" mass is converted into a tremendous amount of energy, according to Einstein's famous equation, E=mc². The energy released manifests as kinetic energy of the fission fragments and as gamma radiation.
Example: The fission of Uranium-235 is a well-known example. When a Uranium-235 nucleus absorbs a neutron, it becomes unstable and splits into lighter nuclei like Krypton and Barium, along with several neutrons. These neutrons can then trigger further fission reactions, leading to a chain reaction—the basis of nuclear reactors and atomic bombs.
The Role of Mass Defect in Fission
The crucial aspect of fission is the mass defect. The total mass of the products (lighter nuclei and neutrons) is slightly less than the mass of the original Uranium-235 nucleus. This difference in mass, even though small, represents a significant amount of energy released according to E=mc². This energy release is what makes fission such a powerful energy source.
Fusion: Joining Nuclei
Nuclear fusion, in contrast to fission, involves the merging of two light nuclei to form a heavier nucleus. This process typically requires extremely high temperatures and pressures to overcome the electrostatic repulsion between the positively charged nuclei. Once the nuclei are close enough, the strong nuclear force takes over, binding them together. Again, the mass of the resulting nucleus is less than the sum of the masses of the original nuclei. This mass difference is converted into energy, often in the form of kinetic energy of the fusion products and electromagnetic radiation.
Example: The fusion of hydrogen isotopes, deuterium and tritium, into helium is a prime example of fusion. This reaction releases a vast amount of energy, forming the basis of the energy production in stars, including our Sun.
The Energy Output of Fusion
Fusion reactions release even more energy per unit mass than fission reactions. This immense energy release stems from the significantly larger mass defect in fusion processes. The high energy output and relatively clean byproducts of fusion make it an extremely attractive source of energy for the future, although harnessing this energy on a large scale presents significant technological challenges.
Mass-Energy Equivalence: E=mc² Explained
Einstein's famous equation, E=mc², elegantly expresses the equivalence of mass and energy. It states that energy (E) and mass (m) are interchangeable, related by the speed of light (c) squared. This equation is fundamental to understanding the energy released in nuclear reactions. The "c²" term is a huge number (approximately 9 x 10¹⁶ m²/s²), emphasizing that even a small amount of mass conversion results in a colossal amount of energy.
Applying E=mc² to Nuclear Reactions
In both fission and fusion, the mass of the products is less than the mass of the reactants. This difference in mass (Δm) is converted into energy (ΔE) according to E=mc². This energy is released as kinetic energy of the particles produced and as electromagnetic radiation (gamma rays).
Calculating Energy Release: To calculate the energy released in a nuclear reaction, one needs to determine the mass defect (Δm) and then apply Einstein's equation. However, the calculations require careful consideration of units and conversion factors, often using atomic mass units (amu) and converting to Joules.
Beyond Fission and Fusion: Other Nuclear Processes
While fission and fusion are the most prominent examples of nuclear reactions involving matter conversion, other processes contribute to this phenomenon.
Radioactive Decay
Radioactive decay involves the spontaneous transformation of an unstable nucleus into a more stable configuration. This transformation can involve the emission of alpha particles (helium nuclei), beta particles (electrons or positrons), or gamma rays (high-energy photons). Each decay process involves a small mass defect, which is converted into energy according to E=mc². The energy released is characteristic of the specific radioactive isotope and its decay mode.
Nuclear Reactions in Stars
Nuclear fusion is the primary energy source in stars. In the Sun's core, hydrogen nuclei fuse to form helium, releasing immense amounts of energy in the process. This fusion process, specifically the proton-proton chain reaction, converts a tiny fraction of the Sun's mass into energy, sustaining its luminosity for billions of years. The energy produced eventually reaches the Earth as sunlight, powering life and influencing weather patterns.
Applications and Implications of Matter-Energy Conversion
The conversion of matter into energy in nuclear reactions has far-reaching implications, impacting various aspects of human society.
Nuclear Power Generation
Nuclear fission is currently used in nuclear power plants to generate electricity. The heat generated from controlled fission reactions is used to boil water, producing steam that drives turbines connected to generators, ultimately generating electricity. This method provides a significant portion of electricity in several countries.
Nuclear Weapons
Uncontrolled nuclear fission chain reactions form the basis of atomic bombs. The immense energy released in a short time frame causes devastating destruction and long-term environmental consequences. Nuclear fusion reactions, though significantly more challenging to control, also hold the potential for even more powerful weapons.
Medical Applications
Radioactive isotopes are used in various medical applications, including diagnosis (e.g., PET scans) and treatment (e.g., radiotherapy). The radiation emitted from these isotopes can be used to image internal organs or to destroy cancerous cells. The energy released from these decay processes is carefully controlled and used for therapeutic purposes.
Future Directions and Challenges
Ongoing research continues to explore various aspects of matter-energy conversion in nuclear reactions.
Fusion Power
Harnessing fusion energy remains a major goal for scientists and engineers worldwide. Developing practical and efficient fusion reactors could provide a clean, safe, and virtually limitless source of energy for future generations. However, overcoming the enormous technological challenges associated with achieving and sustaining the required conditions for fusion remains a significant hurdle.
Advanced Nuclear Reactors
The development of advanced nuclear reactor designs aims to improve safety, efficiency, and waste management in nuclear fission power generation. These designs include strategies to minimize the production of long-lived radioactive waste and to improve the overall sustainability of nuclear power.
Conclusion
The conversion of matter into energy in nuclear reactions is a fundamental process with far-reaching implications for humanity. Understanding the intricacies of nuclear forces, fission, fusion, and radioactive decay provides insight into the immense power locked within the atom. Harnessing this power responsibly and sustainably remains a crucial challenge for the future, with both immense potential benefits and significant risks to consider. Continued research and technological advancements are crucial to harnessing the benefits of nuclear reactions while mitigating potential risks, ensuring a secure and sustainable energy future.
Latest Posts
Latest Posts
-
A Punnett Square Is Used To
Apr 19, 2025
-
How Do Activators And Repressors Affect Transcription
Apr 19, 2025
-
The First Electron Acceptor Of Cellular Respiration Is
Apr 19, 2025
-
Glycolysis Cannot Occur In An Anaerobic Environment
Apr 19, 2025
-
Can A Quadratic Function Be Periodic
Apr 19, 2025
Related Post
Thank you for visiting our website which covers about When Matter Is Converted In A Nuclear Reaction . We hope the information provided has been useful to you. Feel free to contact us if you have any questions or need further assistance. See you next time and don't miss to bookmark.